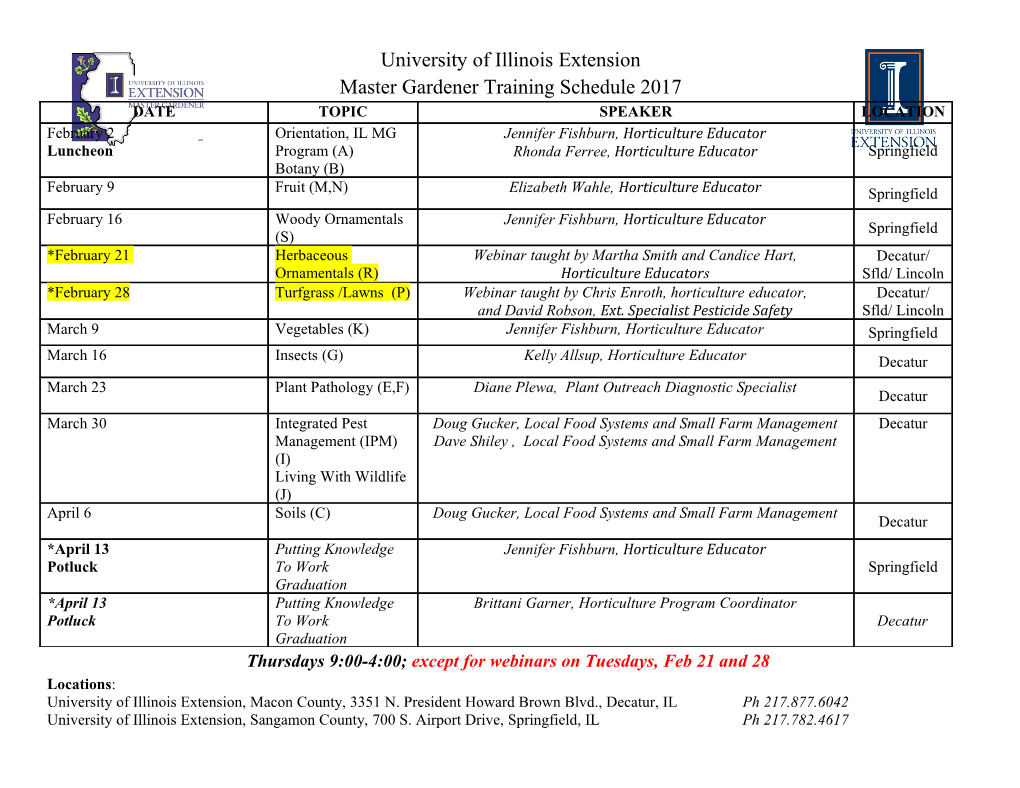
1 2 3 ON THE SPECTRA LUMINESCENCE EMISSION OF 4 NATURAL COAL AND GRAPHITE SPECIMENS 5 6 Irena Kostovaa, Laura Tormob, Elena Crespo-Feoc, Javier Garcia-Guineab, 7 8 aSofia University “St Kliment Ohridski”, Department of Geology and Paleontology, Sofia 1000, Bulgaria 9 bMuseo Nacional Ciencias Naturales, CSIC. C/José Gutiérrez Abascal 2. Madrid 28006, Spain. 10 cFacultad de Ciencias Geologicas. C/Antonio Novais 2. Madrid 28040, Spain. 11 Author for Correspondence: [email protected] 12 13 ABSTRACT 14 The little luminescence shown by coals has been attributed to accessorial minerals and 15 poly-nuclear aromatic hydrocarbons, such as exinite, vitrinite or inertinite, while the 16 luminescence quenching has been found in coal-hydrogenation asphaltenes and 17 pyridine extracts. Nowadays, the spatial resolution and the improved luminescence 18 efficiency of the modern spectrometers allow explain some details on the luminescent 19 emission centers in the same theoretical frame. Accordingly, we selected Museum 20 historical coals specimens with different rank, i.e., peat, lignite, sub-bituminous, 21 bituminous, anthracite, to be analyzed by the spectra cathodoluminescence probe (CL) 22 of an environmental scanning electron microscopy (ESEM) with an energy dispersive 23 spectrometry analyzer (EDS). Additional analytical controls were also performed by X- 24 ray diffraction (XRD), X-ray fluorescence (XRF) and Raman spectrometries. We 25 conclude that coals may display different luminescence emission features from several 26 sources, as follows: (i) broad band of intense luminescence from poly-nuclear aromatic 27 hydrocarbons, (ii) little visible broadband luminescence attributed to band-tail states 28 caused by variations in the energy gap of individual sp2 carbon clusters due to their 29 difference in size and/or shape, (iii) silicate impurities as responsible of the common 30 luminescence peak at 325nm observed in coals attributed to non-bridging oxygen hole 31 centres (≡ Si–O•) probably generated from precursor Si–O–C species formed by ≡ Si– 32 O• defects and carbon atoms; (iv) a 710 nm CL emission commonly detected also in 33 wood and ivories which has been correlated with hydrocarbon groups of chlorophyll or 34 lignine. Coals are very complex rocks composed by both organic and inorganic phases 35 with variable and complex spectra needing more analyses together with carbonous 36 standards of graphite, silicon carbide, stuffed carbon silica and diamond at variable 37 experimental conditions. 38 Keywords: Luminescence, Cathodoluminescence, Raman, NBOHC, Coal, Graphite, 39 40 41 INTRODUCTION 42 The increasing use of the Spectra Cathodoluminescence (CL) and X-ray Energy 43 Dispersive Spectroscopy (EDS) probes coupled to environmental electron microscopes 44 (ESEM) for geological materials analyses suggest us to enquire on the frequent 45 observed spectra CL of nominally non-luminescent natural carbonous compounds such 46 as graphite and coals. The new devices such as the MONOCL3 Gatam probe records 47 CL spectra at high resolution by a retractable parabolic diamond mirror and 1 48 photomultiplier tube which collect and amplify the CL emission with great quantum 49 efficiency. These new spatially-resolved facilities could provide accurate spectral 50 details not previously observed in non-luminescent materials. Accordingly, it seems 51 important to clarify feasible causes of CL emissions in these carbonous compounds 52 exhibiting low luminescence. From the sixties decade it is well known that the little 53 visible luminescence shown by coals, if any, come up from accessorial minerals in 54 coals such as feldspar, quartz, calcite, gypsum and kaolinite. In addition, coals with 55 large amounts of poly-nuclear aromatic hydrocarbons (PAH) as well as large amounts 56 of free radicals should exhibit an appreciable visible luminescence (1). The lack of 57 luminescence in solid coals or solid derivatives, such as coal-hydrogenation 58 asphaltenes and pyridine extracts, could be explained by quenching due to various 59 factors. Carbon-disulphide extracts of coal macerals, e.g., exinite, vitrinite, inertinite, 60 exhibit luminescence emission which can be employed to identify individual polycyclic 61 hydrocarbons (2). Recently, many other natural hydrocarbons and resins associated to 62 coals, slates or peatified woods, e.g. such as hatchettite, fichtelite, hartite, ozokerite are 63 being studied by spectroscopy, since they exhibit very different and complex Raman 64 spectra (3,4). Early comparative studies on the Raman and Luminescence spectra in 65 coal and graphite show large similarities among coal and disordered graphite -1 66 suggesting that the Raman peak near 1600 cm originates from the zone-center E2g -1 67 mode of graphite and the assignment of the peak around 1370 cm for disordered 68 graphite as the A1g mode of D6h (5). The Raman spectra consist of two main bands at 69 1600 and 1360 cm-1 which usually have bandwidths at half peak intensity depending on 70 the rank of the coal. The origin of these bands is best discussed in relation to those -1 71 found in graphites and carbons. The band at 1600 cm has been interpreted as being -1 72 related to the 1580 cm band of graphite which has been assigned to the E2g carbon- 73 carbon in-plane stretching vibration. The Raman band at 1360 cm-1 has been attributed 74 to defects present in structural units and disorder (6). Water and hydroxyl groups 75 commonly integrated in coals and associated clay minerals must be considered 76 analyzing dehydration induced luminescence, e.g., thermoluminescence (TL) or CL. 77 Both techniques produce tribo-luminescence phenomena during the luminescence 78 measurements, i.e., extensive mechanical distortion produced on freezing and drying in 79 a wet/dry cycled reaction sequence (7,8). Water and coupled hydro-silicate molecules 80 could be involved in the thermal stimulation processes to obtain luminescence 81 emission from natural coals. An intense ultraviolet and green photoluminescence can 82 be observed from sol–gel derived silica containing hydrogenated carbon (9). The 83 intensity of the UV PL 330 nm emission increases significantly with the increase of OH 84 groups adsorbed on the surface of porous silica since strain Si–O bonds creating 85 silicon vacancy-hole centers, Si–O bonding defects seem to be responsible of the 86 NBOHC, non-bridging oxygen hole centres ≡( Si –O•) for the 340nm emission (10). 87 Concerning silica in coals, detritic and diagenetic quartz and amorphous silica phases 88 are frequently found in natural coals (11); the integrated SEM-cathodoluminescence 89 systems are being used to classify quartz grains in coals on the basis of their origin, 90 i.e., metamorphic, igneous, hydrothermal (12) but these spectra UV luminescence 91 bands are commonly overlooked. Diamond and graphite carbon—carbon structures 92 show different characteristic luminescence broad bands, observed for diamonds 93 peaked ca 617 nm due to electron-lattice interaction of the nitrogen centers (13). 94 Graphites show signs of a perceptible broadband luminescence explained by band-tail 95 states produced by changes in the energy gap of individual sp2 carbon clusters by 2 96 differences in size and/or shape (14). This paper focuses on the luminescence 97 emissions of carbonous compounds studied by EDS, ESEM XRF, and CL. For 98 comparison, we also perform Raman spectroscopy as a consolidated technique used 99 to check metamorphism and maturation grade of coals (15). 100 101 MATERIALS AND EXPERIMENTAL METHODS 102 We select aliquots of 9 historical-international coals kept in the reserve of the MNCN 103 museum (Madrid, Spain) with the following data-labeling: A2 Hartite (C20H34) from 104 Gloggnitz (Vienne, Austria), A3 Lignite from Vienne, GB1 Coal from Cardiff (UK), GB3 105 Elaterite (Bitumen) from Derbyshire (UK), CS2 Lignite from Bohemia (Czec Rep.), NL1 106 Peat from Amsterdam, SU1 Shungite (C60) from Olensk, Gon, Russia, USA1 Anthracite 107 from Pennsylvania, TR1 Anthracite from Armenia (Turkey). In addition, we also select 108 standards of graphite from Sri Lanka, black SiC with graphite, green SiC of Navarro 109 S.A. and synthetic diamond Nanyang Zhongnan Diamond Co. of China. Some aliquots 110 were pre-heated at different time-temperature sets from 100ºC to 600ºC in both, 111 oxidant-atmospheric and reductor environments. In the graphite aliquots case, different 112 crystallographic orientations (010) and (001) were also prepared for the luminescence 113 measurements. Qualitative, quantitative and micro-textural XRD analyses of powdered 114 coal aliquots were performed using XPOWDER software which also allows a full duplex 115 control of the Philips PW-1710/00 diffractometer using the CuKα radiation with a Ni 116 filter and a setting of 40kV and 40mA. The qualitative search-matching procedure was 117 based on the ICDD-PDF2 database and the DIFDATA free database of the RRUFF 118 Project. We utilize Boolean searching and chemical restraints to the initial elements 119 previously detected by X-ray fluorescence spectrometry. Chemical XRF analyses of 120 coals were performed in a Magic Philips X-ray fluorescence spectrometer operating an 121 ultra-thin window and rhodium anode X-ray tube at 2.4 kW. The equipment has two 122 coupled flux and sparkling detectors in the spectrometric chamber. In addition, there 123 are three collimators of 150, 300 and 700μm for high resolution, quantitative analysis, 124 and light elements analysis. Five analyser crystals, LiF 220, LiF 200, Ge, PE and Px1, 125 allow the detection of all the usual chemical elements from oxygen to uranium. The 126 coal powder pellets (trace elements) were mounted using 8.0 g of sample and 3.5 ml of 127 elvacite with 20% acetone all together pressed at 200 KNcm-2. The ESEM XL30 128 microscope of FEI Company is a facility sited in the MNCN Museum. It is a low-vacuum 129 ESEM which enables high-resolution inspection and chemical analysis of non- 130 conductive specimens. The ESEM microscope in low vacuum mode admits hydrated 131 samples to be studied in their original state with the Large Field Detector (LFD), since it 132 is close to the sample to avoid electron losses.
Details
-
File Typepdf
-
Upload Time-
-
Content LanguagesEnglish
-
Upload UserAnonymous/Not logged-in
-
File Pages18 Page
-
File Size-