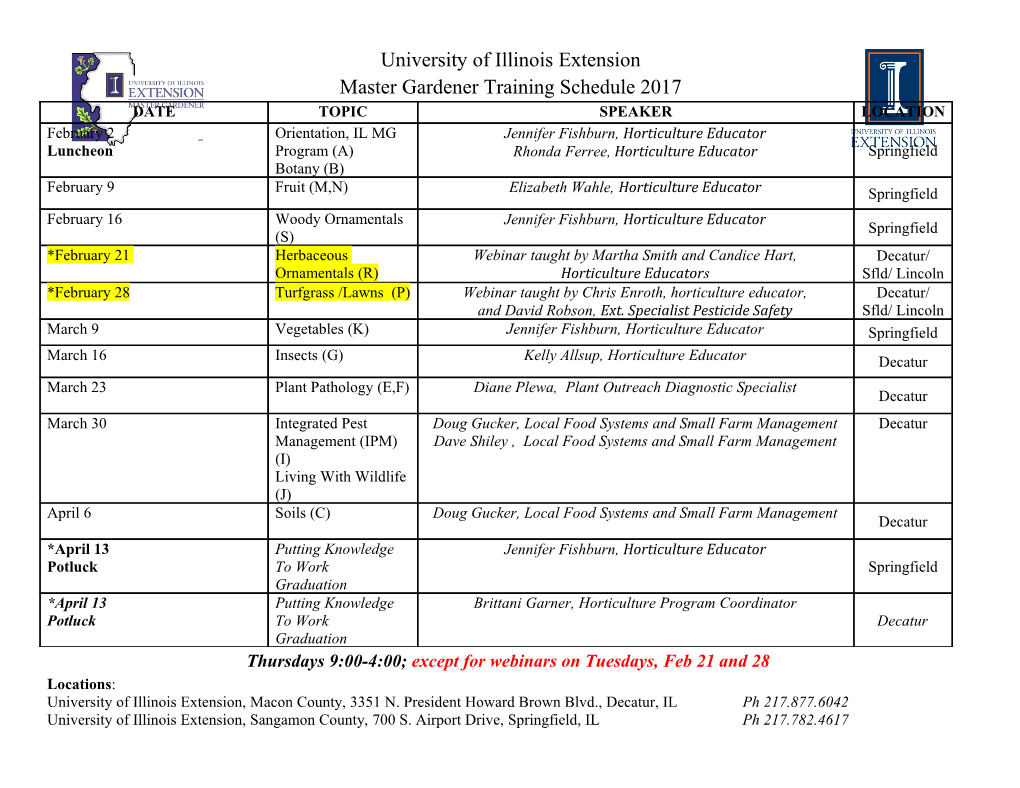
Evaluation of SAS1B as an Immunotherapeutic Target for the Treatment of Cancer Kiley Anderson Knapp Barre, Vermont Bachelor of Science, University of New Hampshire, 2009 A Dissertation Presented to the Graduate Faculty of the University of Virginia in Candidacy for the Degree of Doctor of Philosophy Experimental Pathology University of Virginia May, 2018 ii Dedication I dedicate my work to daughter, Harper, my husband, Brook, and to my parents Kristin and Michael Gilbar and Thomas and Holly Anderson. My successes are owed in large part to my family; I sincerely thank them for their continued unconditional love and support. iii Acknowledgements Firstly, I offer thanks to my late mentor, Dr. John C. Herr (1948-2016), for the many lessons learned not only in science but also in leadership, perseverance, and expert- level gardening. For those of us working in Dr. Herr’s lab, his passion for and dedication to his research served as regular inspiration and motivation. His vision and expertise inspired this work and I am grateful to have had the opportunity to work with him. I also thank Dr. Timothy Bullock for serving as an exceptional mentor to me after Dr. Herr’s passing. I am grateful that despite his busy schedule, Dr. Bullock was regularly available and willing to offer guidance. Dr. Bullock went above and beyond with regard to intellectual support, research guidance, and invaluable assistance with manuscript and dissertation preparation for which I thank him. Dr. Eusebio Pires has also been a great mentor and friend of mine throughout this work and I am grateful to him for that. I thank Dr. Pires for patiently teaching me, answering my relentless questions, and for the endless discussions. Many of the protocols utilized in this work were adapted from Dr. Pires’ previous work. Dr. Pires’ general scientific support was crucial to this research. I also thank the following members of my dissertation committee: Dr. James Mandell, Dr. Timothy Bullock, Dr. J. Thomas Parsons, Dr. Janet Cross, Dr. Amy Bouton, Dr. Charles Landen, and Dr. Barry Hinton for their continued support and scientific counseling. I am grateful for the discussions with and suggestions from the committee which helped guide the research aims and the progress of this work. A number of people contributed experimentally to this work whom I would also like to thank. Dr. Pires performed the experiments represented in Supplemental Figure 2.1. iv Dr. Walter Olson and Aaron Stevens performed the work shown in Figures 3.2 and 3.3, respectively. Dr. Jagathalpa Shetty performed the experiments in Figure 3.11. Dr. Michael Harding contributed the hypothesis regarding SAS1B’s active role as a metalloprotease which cleaves ZP domain containing proteins in cancer. I would like to thank Dr. Scott Vande Pol and Nicole Brimer at UVA for providing neonatal keratinocytes and offering support for cell growth and expansion. I am grateful to Dr. Jagathpala Shetty, Dr. Ryan D’Souza, and Dr. Michael Harding, and Dr. Arabinda Mandal for continued general intellectual support and guidance. I also thank the members of the following UVA Core Facilities: the Research Histology Core, the Flow Cytometry Core, the Cell Culture core, the Microscopy Facility, and the Biorepository and Tissue Research Facility. v Abstract Immunotherapeutic options for the treatment of cancer, which remains a major global health challenge, offer the allure of greater tumor specificity and less associated toxicity than is typically achieved with traditional chemo- and radio-therapeutic strategies. The success of some immunotherapies, such as ADCs and CAR-Ts, rely on identification and selection of targets which are highly tumor-specific with limited or no expression in normal tissues. Cancer germline antigens represent potential ideal targets for targeted immunotherapy as CGAs are expressed in cancer cells but show limited or no expression among normal tissues. Although many cancer-testis antigens have been described, SAS1B is the first, and only, cancer-oocyte antigen identified to-date. Owing to the limited expression of SAS1B among normal tissue combined with expression in a number of cancer indications, we propose that SAS1B is an attractive immunotherapeutic target. We have shown that, in addition to previous work published in female reproductive cancers, SAS1B is expressed in a majority of pancreatic and head and neck cancers. SAS1B localized to both the cytoplasm and the cell surface in PDAC and HNSCC cell lines by IIF and flow cytometry, suggesting potential utility of SAS1B targeted immunotherapeutic strategies. Furthermore, an ADC targeting SAS1B administered to pancreatic cancer cell lines was internalized and subsequently caused significant cell death in a manner correlated with SAS1B cell surface expression. Thus, SAS1B represents a novel therapeutic target for the treatment of PDAC and HNSCC. These data support further development of a SAS1B-ADC including in vivo assessment using mouse xenograft systems. vi Although we have shown proof of concept that SAS1B-ADC induces cytotoxicity in pancreatic cancer cell lines, addressing multiple fundamental biological questions which remain regarding SAS1B expression will also inform production of SAS1B targeted therapies. For example, we have identified six ASTL splice variants in cancer, known as SV-A to SV-F, and have shown differential cellular localization of recombinant SV-A and SV-C proteins (cell surface vs. cytoplasm, respectively). Further studies characterizing major SAS1B protein isoform(s) expressed at the cell surface in cancers may lead to development of more effective immunotherapies utilizing mAbs generated against cell surface, cancer-associated form(s) of SAS1B. Our work suggests SAS1B expression in a broad range of cancer indications and demonstrates efficacy of a SAS1B-ADC in vitro, thus supporting further assessment of SAS1B as an immunotherapeutic target for the treatment of cancer. 1 Table of Contents List of Figures……………………………………………………………………………..3 Chapter 1: General Introduction Cancer: Statistics and Common Therapeutic Options…………………………….6 Select Immunotherapeutic Options for Cancer Treatment………………………..7 Antibody Drug Conjugates……………………………………………………….10 SAS1B…………………………………………………………………………... 14 Astacin Family of Metalloendopeptidases……………………………………….17 Tumor Neoantigens……………………………………………………………....19 Chapter 2: Evaluation of SAS1B as a target for antibody-drug conjugate therapy in the treatment of pancreatic cancer (modified from Oncotarget submission) Abstract…………………………………………………………………………..23 Introduction………………………………………………………………………24 Results……………………………………………………………………………26 Discussion………………………………………………………………………..46 Methods………………………………………………………………………….53 Chapter 3: ASTL/SAS1B: Expression in normal cells and additional cancers Introduction………………………………………………………………………63 Results……………………………………………………………………………69 Discussion………………………………………………………………………105 Methods…………………………………………………………………………121 2 Chapter 4: Future Directions What is the pattern of expression and cellular localization of ASTL splice variants and SAS1B isoforms in cancer…………………………………………………130 What is the functional role of SAS1B in cancer…………………………………132 How is SAS1B regulated in cancer……………………………………………..138 Is SAS1B expressed in pancreatic cancer stem cells…………………………….141 What is the threshold of SAS1B surface expression needed for ADC cytotoxicity in PDAC cell lines and how does this relate to potential cutoff for which patients will be treated……………………………………………………………….…..143 Does a SAS1B-ADC effectively stop tumor growth or shrink tumors in vivo…………………………………………………………………………..….145 Final Summary………………………………………………………………………….148 Chapter 5: References………………………………………..…………………..……..152 3 List of Figures Figure 1.1: SAS1B protein domain structure……………………………………………16 Figure 2.1: SAS1B was expressed in a majority of pancreatic cancers and was not detected in normal pancreas ductal epithelium by IHC………………………………….29 Figure 2.2: SAS1B signal in pancreatic cancer tissue was blocked by pre-incubating anti- SAS1B mAb, 6B1, with rSAS1B…………………………………………………..........31 Figure 2.3: ASTL/SAS1B expression in pancreatic cancer patient derived xenografts...32 Figure 2.4: SAS1B localized to the cytoplasm and to the cell surface in pancreatic cancer cell lines.…………………………………………………………………………………35 Figure 2.5: SAS1B surface expression in pancreatic cancer cell lines correlated to anti- SAS1B ADC cell killing in vitro………………………………………...………………41 Figure 2.6: Anti-SAS1B mAb, SB2, signal in live mPanc96 cells was blocked when pre- incubated with rSAS1B protein………………………………………………………….42 Figure 2.7: ADCs with different anti-SAS1B mAbs are also cytotoxic to mPanc96 cells………………………………………………………………………………………43 Figure 2.8: Anti-SAS1B mAb, SB2, alone was not cytotoxic to pancreatic cancer cell lines……………………………………………………………………………………....44 Figure 2.9: Cytotoxicity of an anti-SAS1B ADC was blocked in pancreatic cancer cell lines when SB2 mAb was pre-incubated with a SAS1B peptide………………………..45 Supplemental Figure 2.1: SAS1B mAb 6B1 preferentially recognizes cytoplasmic SAS1B…………………………………………………………………………………...60 Figure 3.1: ASTL gene expression data from GTEx database………………………….70 Figure 3.2: SAS1B localizes to the cytoplasm, but not to the cell surface, in a small population of human PBMCs……………………………………………………………73 Figure 3.3: Mouse SAS1B was expressed intracellularly in dendritic cells…………….76 4 Figure 3.4: ASTL/SAS1B was expressed in a high percentage of patient HNSCCs…...81 Figure 3.5: HNSCC cell lines expressed ASTL/SAS1B………………………………..82
Details
-
File Typepdf
-
Upload Time-
-
Content LanguagesEnglish
-
Upload UserAnonymous/Not logged-in
-
File Pages174 Page
-
File Size-