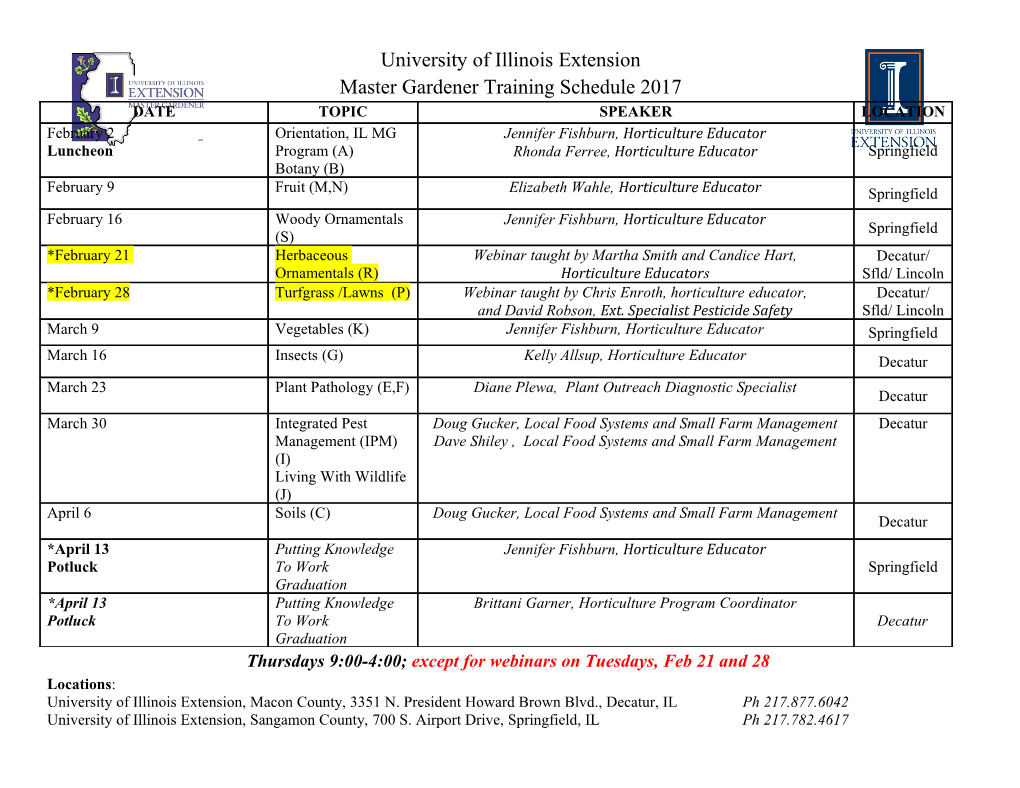
UP-DOWN ORDERED CHINESE RESTAURANT PROCESSES WITH TWO-SIDED IMMIGRATION, EMIGRATION AND DIFFUSION LIMITS BY QUAN SHI1 AND MATTHIAS WINKEL2 1Mathematical Institute, University of Mannheim, Mannheim D-68131, Germany. [email protected] 2Department of Statistics, University of Oxford, 24–29 St Giles’, Oxford OX1 3LB, UK. [email protected] We introduce a three-parameter family of up-down ordered Chinese (α) restaurant processes PCRP (θ1; θ2), α 2 (0; 1), θ1; θ2 ≥ 0, generalising the two-parameter family of Rogers and Winkel. Our main result establishes (α) self-similar diffusion limits, SSIP (θ1; θ2)-evolutions generalising exist- ing families of interval partition evolutions. We use the scaling limit approach to extend stationarity results to the full three-parameter family, identifying an extended family of Poisson–Dirichlet interval partitions. Their ranked se- quence of interval lengths has Poisson–Dirichlet distribution with parameters α 2 (0; 1) and θ := θ1 + θ2 − α ≥ −α, including for the first time the usual range of θ > −α rather than being restricted to θ ≥ 0. This has applications to Fleming–Viot processes, nested interval partition evolutions and tree-valued Markov processes, notably relying on the extended parameter range. 1. Introduction. The primary purpose of this work is to study the weak convergence of a family of properly rescaled continuous-time Markov chains on integer compositions [22] and the limiting diffusions. Our results should be compared with the scaling limits of natural up-down Markov chains on branching graphs, which have received substantial focus in the literature [8, 35, 36]. In this language, our models take place on the branching graph of integer compositions and on its boundary, which was represented in [22] as a space of interval partitions. This paper establishes a proper scaling limit connection between discrete models [44] and their continuum analogues [14, 15, 18] in the generality of [47]. We consider a class of ordered Chinese restaurant processes with departures, parametrised by α 2 (0; 1) and θ1; θ2 ≥ 0. This model is a continuous-time Markov chain (C(t); t ≥ 0) on vectors of positive integers, describing customer numbers of occupied tables arranged in a row. At time zero, say that there are k 2 N = f1; 2;:::g occupied tables and for each i ≤ k the i-th occupied table enumerated from left to right has ni 2 N customers, then the initial state is C(0) = (n1; : : : ; nk). New customers arrive as time proceeds, either taking a seat at an existing table or starting a new table, according to the following rule, illustrated in Figure1: • for each occupied table, say there are m 2 N customers, a new customer comes to join this table at rate m − α; arXiv:2012.15758v1 [math.PR] 31 Dec 2020 • at rate θ1, a new customer enters to start a new table to the left of the leftmost table; • at rate θ2, a new customer begins a new table to the right of the rightmost table; • between each pair of two neighbouring occupied tables, a new customer enters and begins a new table there at rate α. We refer to the arrival of a customer as an up-step. Furthermore, each customer leaves at rate 1 (a down-step). By convention, the chain jumps from the null vector ; to state (1) at rate θ := θ1 + θ2 − α if θ > 0, and ; is an absorbing state if θ ≤ 0. At every time t ≥ 0, let C(t) MSC2020 subject classifications: Primary 60J80; secondary 60C05, 60F17. Keywords and phrases: Poisson–Dirichlet distribution, interval partition, Chinese restaurant process, integer composition, self-similar process, branching with immigration and emigration. 1 2 Q. SHI AND M. WINKEL (α) FIG 1. The rates at which new customers arrive in a PCRP (θ1; θ2). be the vector of customer numbers at occupied tables, listed from left to right. In this way we have defined a continuous-time Markov chain (C(t); t ≥ 0). This process is referred to as a Poissonised up-down ordered Chinese restaurant process (PCRP) with parameters α, θ1 and (α) θ2, denoted by PCRPC(0)(θ1; θ2). This family of Markov chains is closely related to the well-known Chinese restaurant processes due to Dubins and Pitman (see e.g. [38]) and their ordered variants studied in (α) [26, 39]. When θ2 = α, a PCRP (θ1; α) is studied in [44]. Notably, our generalisation includes cases θ = θ1 + θ2 − α 2 (−α; 0), which did not arise in [26, 39, 44]. Though we focus on the range α 2 (0; 1) in this paper, our model is clearly well-defined for α = 0 and it is straightforward to deal with this case; we include a discussion in Section 4.7. To state our first main result, we represent PCRPs in a space of interval partitions. For M ≥ 0, an interval partition β = fUi; i 2 Ig of [0;M] is a (finite or countably infinite) collection of disjoint open intervals Ui = (ai; bi) ⊆ (0;M), such that the (compact) set of partition points S G(β) := [0;M] n Ui has zero Lebesgue measure. We refer to the intervals U 2 β as i2I P blocks, to their lengths Leb(U) as their masses. We similarly refer to kβk := U2β Leb(U) as the total mass of β. We denote by IH the set of all interval partitions of [0;M] for all M ≥ 0. This space is equipped with the metric dH obtained by applying the Hausdorff metric 0 to the sets of partition points: for every γ; γ 2 IH , 0 [ 0 [ dH (γ; γ ) := inf r ≥ 0: G(γ) ⊆ (x − r; x + r);G(γ ) ⊆ (x − r; x + r) : x2G(γ0) x2G(γ) Although (IH ; dH ) is not complete, the induced topological space is Polish [16, Theo- rem 2.3]. For c > 0 and β 2 IH , we define a scaling map by cβ := f(ca; cb):(a; b) 2 βg: We shall regard a PCRP (C(t); t ≥ 0) as a càdlàg process in (IH ; dH ), by identifying any vector of positive integers (n1; : : : ; nk) with an interval partition of [0; n1 + ··· + nk]: (n1; : : : ; nk) ! f(si−1; si); 1 ≤ i ≤ kg where si = n1 + ··· + ni: We are now ready to state our main result, which is a limit theorem in distribution in the space of IH -valued càdlàg functions D(R+; IH ) with R+ := [0; 1), endowed with the J1- Skorokhod topology (see e.g. [6] for background). (n) THEOREM 1.1. Let α 2 (0; 1) and θ1; θ2 ≥ 0. For n 2 N, let (C (t); t ≥ 0) be a (α) (n) (n) PCRP (θ1; θ2) starting from C (0) = γ . Suppose that the initial interval partitions 1 (n) n γ converge in distribution to γ 2 IH as n ! 1, under dH . Then there exists an IH - valued path-continuous Hunt process (β(t); t ≥ 0) starting from β(0) = γ, such that 1 (n) (1) C (2nt); t ≥ 0 −! (β(t); t ≥ 0); in distribution in D(R+; IH ). n n!1 Moreover, set ζ(n) = infft ≥ 0: C(n)(t) = ;g and ζ = infft ≥ 0: β(t) = ;g to be the respec- tive first hitting times of ;. If γ 6= ;, then (1) holds jointly with ζ(n)=2n ! ζ, in distribution. TWO-SIDED UP-DOWN CRPS AND THEIR DIFFUSION LIMITS 3 We call the limiting diffusion (β(t); t ≥ 0) on IH an (α; θ1; θ2)-self-similar interval par- (α) tition evolution, denoted by SSIP (θ1; θ2). These processes are indeed self-similar with index 1, in the language of self-similar Markov processes [31], see also [29, Chapter 13]: if (α) −1 (α) (β(t); t ≥ 0) is an SSIP (θ1; θ2)-evolution, then (cβ(c t); t ≥ 0) is an SSIP (θ1; θ2)- evolution starting from cβ(0), for any c > 0. While most SSIP-evolutions have been constructed before [14, 15, 18, 47], in increasing generality, Theorem 1.1 is the first scaling limit result with an SSIP-evolution as its limit. In special cases, this was conjectured in [44]. In the following, we state some consequences and further developments. We relate to the literature in more detail in Section 1.1. We refer to Section 1.3 for applications particularly of the generality of the three-parameter family. Our interval partition evolutions have multiple connections to squared Bessel processes. More precisely, a squared Bessel process Z = (Z(t); t ≥ 0) starting from Z(0) = m ≥ 0 and with “dimension” parameter δ 2 R is the unique strong solution of the following equation: Z t Z(t) = m + δt + 2 pjZ(s)jdB(s); 0 where (B(t); t ≥ 0) is a standard Brownian motion. We refer to [23] for general properties of squared Bessel processes. Let ζ(Z) := infft ≥ 0: Z(t) = 0g be the first hitting time of zero. To allow Z to re-enter (0; 1) where possible after hitting 0, we define the lifetime of Z by ( 1; if δ > 0; (2) ζ(Z) := ζ(Z); if δ ≤ 0: We write BESQm(δ) for the law of a squared Bessel process Z with dimension δ starting from m, in the case δ ≤ 0 absorbed in ; at the end of its (finite) lifetime ζ(Z). When δ ≤ 0, by our convention BESQ0(δ) is the law of the constant zero process. (α) In an SSIP (θ1; θ2)-evolution, informally speaking, each block evolves as BESQ(−2α), independently of other blocks [14, 15]. Meanwhile, there is always immigration of rate 2α between “adjacent blocks”, rate 2θ1 on the left [18] and rate 2θ2 on the right [47].
Details
-
File Typepdf
-
Upload Time-
-
Content LanguagesEnglish
-
Upload UserAnonymous/Not logged-in
-
File Pages47 Page
-
File Size-