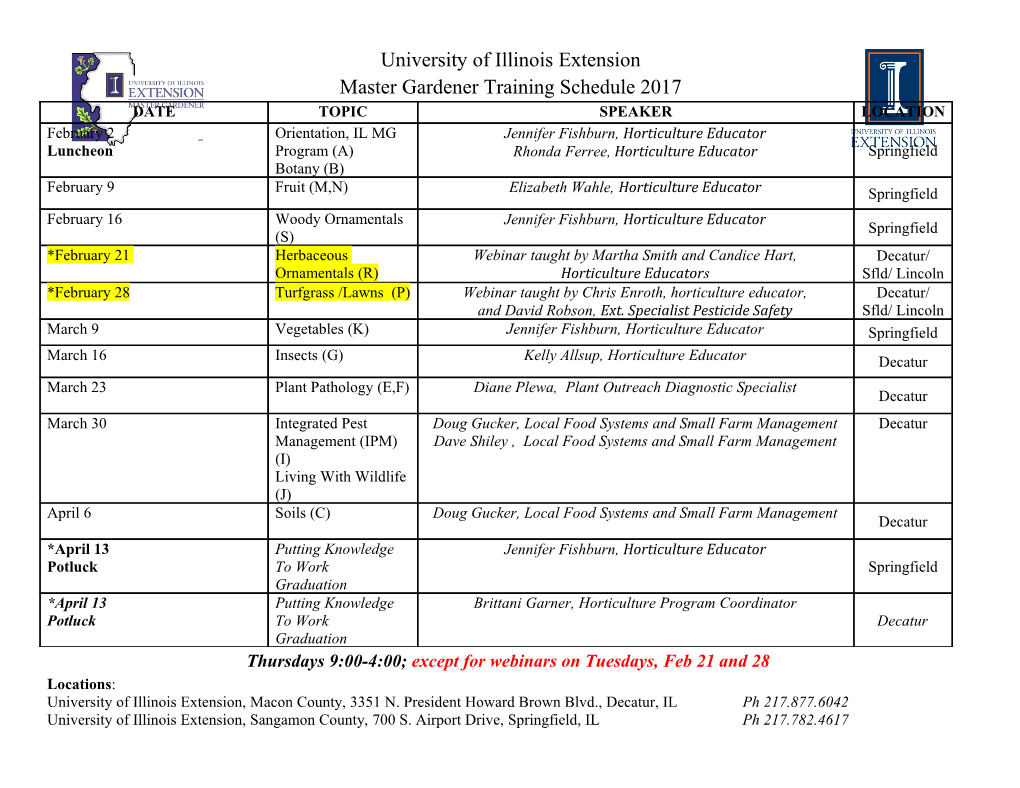
Staehle R., et al. (2013): JoSS, Vol. 2, No. 1, pp. 161-186 (Peer-reviewed Article available at www.jossonline.com) www.DeepakPublishing.com www.JoSSonline.com Interplanetary CubeSats: Opening the Solar System to a Broad Community at Lower Cost Robert L. Staehle1, Diana Blaney1, Hamid Hemmati1, Dayton Jones1, Andrew Klesh1, Paulett Liewer1, Joseph Lazio1, Martin Wen-Yu Lo1, Pantazis Mouroulis1, Neil Murphy1, Paula J. Pingree1, Thor Wilson1, Brian Anderson2, C. Channing Chow II2†, Bruce Betts3, Louis Friedman3, Jordi Puig-Suari4, Austin Williams4, and Tomas Svitek5 1 Jet Propulsion Laboratory, California Institute of Technology, Pasadena, CA USA 2 University of Southern California, Los Angeles, CA USA 3 The Planetary Society, Pasadena, CA USA 4 California Polytechnic University, San Luis Obispo, CA USA 5 Stellar Exploration, Inc., San Luis Obispo, CA USA Abstract Interplanetary CubeSats could enable small, low-cost missions beyond low Earth orbit (LEO). This class is defined as having a mass of <~10 kg, cost of <$30 M, and mission duration of up to five years. Over the coming decade, a stretch of each of six distinct technology areas, creating one overarching architecture, could enable com- paratively low-cost Solar System exploration missions with capabilities far beyond those demonstrated in small satellites to date. The six technology areas are (1) CubeSat electronics and subsystems extended to operate in the interplanetary environment, especially radiation and duration of operation; (2) optical telecommunications to enable very small, low-power uplink/downlink over interplanetary distances; (3) solar sail propulsion to enable high ΔV maneuvering using no propellant; (4) navigation of the Interplanetary Superhighway to enable multiple destinations over reasonable mission durations using achievable ΔV; (5) small, highly capable instrumentation en- abling acquisition of high-quality scientific and exploration information; and (6) onboard storage and processing of raw instrument data and navigation information to enable maximum utility of uplink and downlink telecom capacity, and minimal operations staffing. The NASA Innovative Advanced Concepts (NIAC) program selected Interplanetary CubeSats in 2011 for further investigation, some results of which are reported here for Phase 1. Copyright © A. Deepak Publishing. All rights reserved. JoSS, Vol. 2, No. 1, p. 161 Staehle R., et al. 1. Introduction 2010a), plus electrochromic tips for attitude control. A two-way optical communications terminal occupying Interplanetary CubeSats could enable small, low- 1U is based on JPL laser telecommunications develop- cost missions beyond low Earth orbit (LEO) (Staehle ments, with a link capacity of 1–4 kbps at 2 AU Earth– et al., 2011). This class is defined as having a mass of spacecraft distance. The final 1U is used for spacecraft <~10 kg, cost of <$30M, and mission duration of up to housekeeping -- command and data handling (C&DH), five years. Over the coming decade, a stretch of each of power, attitude determination -- and is based on Cal- six distinct technology areas, creating one overarching Poly CP7 and Jet Propulsion Laboratory (JPL) CubeSat architecture, can enable comparatively low-cost Solar On-board processing Validation Experiment (COVE) System exploration missions with capabilities far be- avionics (Bekker et al., 2011). yond those demonstrated in small satellites to date. Six Though many different missions would be possible technology developments can be integrated to form a with this architecture, the potential missions initially highly versatile Interplanetary CubeSat architecture: considered under NASA Innovative Advanced Con- 1. CubeSat electronics and subsystems extended cepts (NIAC) sponsorship are: and improved from their LEO implementation 1. Mineral Mapping of Asteroids (Small Body Sci- specifications in order to operate in the inter- ence); planetary environment, with particular atten- 2. Solar System Escape Technology Demonstra- tion to surviving increased radiation and dura- tion (Tech Demo); tion of operation; 3. Earth–Sun Sub-L1 Space Weather Monitor 2. Optical telecommunications to enable very com- (Heliophysics and Terrestrial Applications); pact, low-power uplink/downlink over inter- 4. Phobos Sample Return (Planetary Science); planetary distances; 5. Earth–Moon L2 Radio Quiet Observatory (As- 3. Solar sail propulsion to enable major maneuvers trophysics); and and rendezvous with multiple targets using no 6. Out-of-Ecliptic Missions (Heliophysics). propellant; The objectives and technology drivers of each of 4. Navigation of the Interplanetary Superhighway these missions illustrate the broad spectrum of mis- to enable multiple destinations over reasonable sions made possible by advancing the CubeSat state- mission durations with achievable ΔV; of-the-art beyond LEO. 5. Small, highly capable instrumentation (such as a Fundamental to this capability and its low cost is the miniature imaging spectrometer) enabling ac- ability to volumetrically pack the diverse capabilities quisition of high-quality scientific and explora- common to any independent interplanetary spacecraft tion information; and into a CubeSat standard launch container, in this case 6. Onboard storage and processing of raw instru- 6U, that can ride to geostationary Earth orbit (GEO) or ment data and navigation information to en- higher launch energies along with a primary payload. able maximum utility of uplink and downlink Paramount among the spacecraft capabilities is telecom capacity, and minimal operations staff- the ability to operate a useful payload aboard the In- ing. terplanetary CubeSat. While a simple camera might be Interplanetary CubeSats build on existing Earth- adequate to satisfy minimalist objectives, highly com- orbiting CubeSat architecture. Target spacecraft vol - plex instrumentation is possible within 2U volume. As ume is 10 × 20 × 30 cm (6U in CubeSat parlance, where an example existence proof, we examined an imaging 1U = 10 × 10 × 10 cm). 2U are reserved for the pay- spectrometer capable of mapping the surface compo- load, which is mission specific. The solar sail occupies sition of near-Earth asteroids, to which the spacecraft 2U and deploys to form a 6 × 6 m or larger square. The could be propelled by its solar sail. An obvious require- solar sail is based on the Planetary Society/Stellar Ex- ment driven by the payload is to return substantial data ploration LightSail™ (Staehle et al., 2011; Svitek et al., sets. For a given aperture, optical downlinks can deliver Copyright © A. Deepak Publishing. All rights reserved. JoSS, Vol. 2, No. 1, p. 162 Interplanetary CubeSats: Opening the Solar System to a Broad Community at Lower Cost dramatically larger amounts of data than are possible at with independent propulsion and telecommunications, radio frequency (RF), and the technology is finally ap- became plausible only when all the functions of a use- proaching an ability to package within 1U a laser com- ful interplanetary spacecraft could clearly be packaged munications terminal that receives uplink and trans- within a CubeSat launch container. During the fore- mits downlink. For many types of data, including that seeable future, the sum of equipment to provide such acquired by an imaging spectrometer, significant data functionality far from Earth does not appear feasible processing is typically performed in multiple steps on within the original LEO-bound CubeSat volumetric the ground in order to generate a useful information envelopes up to 3U (10 × 10 × 30 cm). This conclu- product. With high computing capacity now available sion became quite different when 6U (Holemans, 2011) in a flyable field-programmable gate array (FPGA) (10 × 20 × 30 cm) envelopes came under serious con- package, initial steps of data processing beyond simple sideration. The first conceived Interplanetary CubeSat image compression can be performed onboard a Cube- volumetric allocation, including independent propul- Sat within a relatively modest power budget, reducing sion and telecommunications, opened the possibility of data downlink requirements by factors of 10 to 100, so numerous missions within the inner Solar System, well this becomes an important element of a high-capability beyond Earth, and without dedicated launch to their mission architecture. specific destination (Staehle et al., 2011): Finally, all the normal “housekeeping” functions of an interplanetary spacecraft must be provided, includ- 1U Spacecraft Housekeeping ing C&DH, power, attitude control, thermal control, as 2U Solar Sail Propulsion (or electric or other high well as the structure to hold everything together and ΔV alternatives) the mechanisms to deploy selected elements. 1U Telecommunications (optical, or RF at lower data rates out to 1+ AU) 2. Technical Approach 2U Scientific or Other Payload 6U Total 2.1 Configuration Variations on this combination are of course pos- Perhaps the greatest advantage for users of the sible, and will likely be optimal for some specific mis- CubeSat standard is the availability of frequent, low- sions. For example, cislunar missions can operate with- cost launches as secondary payloads, using launch in the next two years using proven RF hardware and mass capability not required by the primary payload. tracking techniques. Lower ΔV missions may some- Unlike numerous secondary payloads before them, times be better performed using electric propulsion, CubeSats gain their low-cost advantage because certain taking advantage of the ease with which
Details
-
File Typepdf
-
Upload Time-
-
Content LanguagesEnglish
-
Upload UserAnonymous/Not logged-in
-
File Pages26 Page
-
File Size-