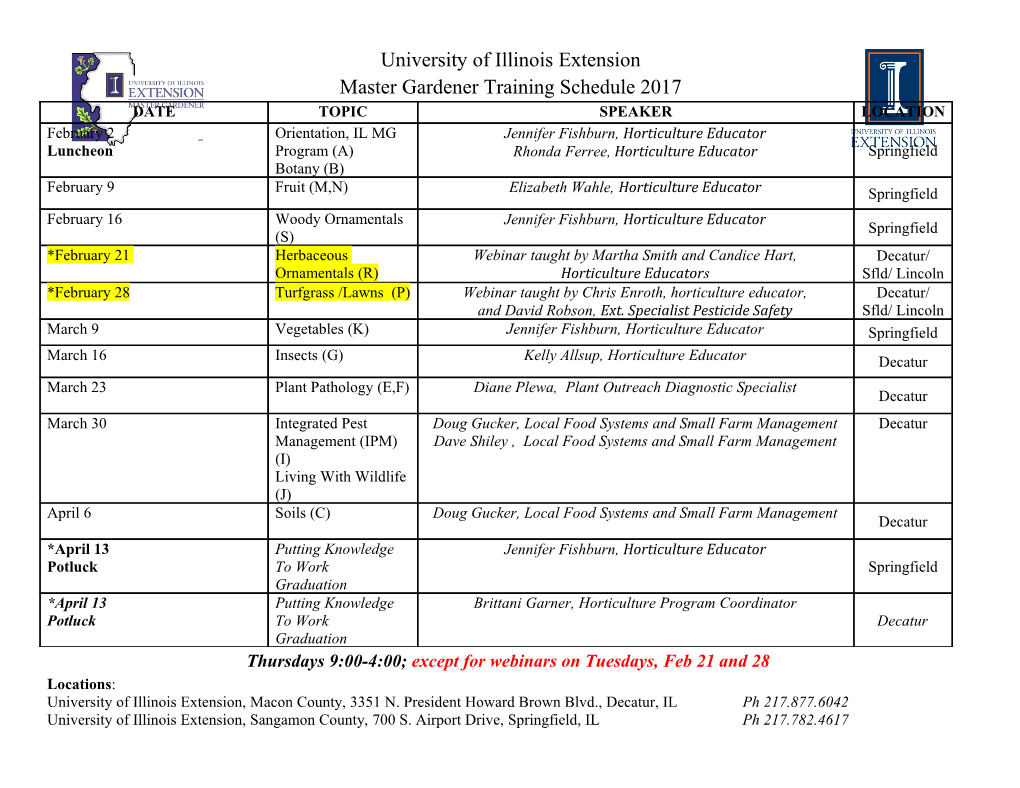
A note on the semisimplicity of group rings 1. (Semi)group rings Let k be any unitary ring (not necessarily commutative), and let S be a semigroup, define kS := fa: S ! k j #Supp(a) 2 Ng (where Supp(a) := fs 2 S j a(s) 6= 0kg) to be the set of all functions from S to k taking only a finite number of non zero values, this is naturally a left k-module and M kS = ks;b where sb: S −! k s.t. sb(x) = δs;x (i.e. sb(x) = 0k if x 6= s; and sb(x) = 1k if x = s ); s2S with respect to this decomposition one can write X X a = (a(s))s2S = a(s)sb = assb (where the sum is actually finite) for any a 2 kS: s2S s2S The k-module kS has also a natural ring structure via the convolution product (it has also the pointwise product, but this is not the one we want), given a; b 2 kS: X ab = a ∗ b is the function defined as ab(s) := a(u)b(v); (u;v)2S×Sjuv=s P P P P P that is ab = ( u2S auub)( v2S avvb) = (u;v)2S×S aubvuvc = s2S( (u;v)2S×Sjuv=s aubv)sb. From this it is not difficult to check that (a; b) 7! ab is associative and distributive. 1.1. Remarks. — Obviously kS is a non zero commutative ring if and only if k is a non zero commutative ring and S is a non empty commutative semigroup. Assume from now on that S is a monoid, i.e. it has a neutral element e 2 S. 1.1.1. Unit. — The element 1keb 2 kS is the unit of the ring kS. Moreover, by definition, k = keb, and S = 1kSb commute each other. 1.1.2. Units. — If G = U(S) is the group (submonoid) of invertible elements in S then U(k)U[(S) = U(k)Gb ⊆ U(kS), where U(·) denotes the set of invertible elements of a unitary ring or monoid. 1.1.3. Decomposable coefficients. — Let k = k1 × k2 be a direct product of two (non trivial) unitary rings. Then 1k = x + y with x; y 2 c(k) two non trivial central and mutually orthogonal idempotents. Then, for any monoid S we get ∼ kS = (k1 × k2)S = k1S × k2S; because 1kS = 1kecS = (x + y)ecS = xecS + yecS with xecS; yecS central and mutually orthogonal idempotents. 1.1.4. Decomposable semigroups. — Let S = S1 × S2 be a direct product of two semigroups, and let k be a unitary ring. Then one can check that ∼ kS = k(S1 × S2) = (kS1)S2: 1.1.5. Group rings. — If S = G is a group, and a; b 2 kG then 0 1 ! X X X X ab = @ aubvA sb = aubu−1s s;b s2S (u;v)2S×Sjuv=s s2S u2S which is the usual form of the convolution product used in Fourier theory. 1 2 1.1.6. Functoriality. — The construction kS is clearly covariantly functorial both in k and in S. Any monoid homomorphism ': S1 −! S2 induces a (unitary) ring homomorphism k': kS1 −! kS2 which is covariantly functorial. And any unitary ring homomorphism f : k1 −! k2 induces a (unitary) ring homomorphism fS : k1S −! k2S which is covariantly functorial. It is not difficult to check that the group ring construction is the left adjoint to the “units” functor from unitary rings to groups; this means that to give a ring homomorphism ': k1G −! k2 where k1 and k2 are rings, and G is a group it is equivalent to give ( a group homomorphism 'jG : G −! U(k2); and a unitary ring homomorphism 'jk1 : k1 −! k2 such that '(k1) ⊆ ck2 ('(G)): 1.1.7. Augmentation (or Norm) and Trace. — Let S be a monoid and k be a unitary ring, we then always have the surjective unitary ring homomorphism, called augmentation, or norm, X X α: kS −! k; assb 7! as which is a retraction of the injective unitary ring homomorphism k −! kS; a 7! aecS: Hence k is a direct summand of kS, indeed kS = k ⊕ Ker(α) for x = α(x) + (x − α(x)) 2 k ⊕ Ker(α). Obviously, for any s 2 S the element sb− eb is inside the two-sided ideal Ker(α), hence Ker(α) contains the two-sided ideal (fsb− eb j s 2 Sg), and actually Ker(α) = (fsb− eb j s 2 Sg) being X X X X X x = assb = assb− 0 = assb− ( as)eb = as(sb− eb) P for any x = assb 2 Ker(α). If S = G is a group, and A ⊆ G generates G as a group then (fgb − eb j g 2 Gg) = (fgb − eb j −1 −1 g 2 Ag) =: IA in kG, indeed if g 2 A then gd − eb = −gd(gb − eb) 2 IA, and if g1; g2 2 A then −1 d−1 −1 gb2 − gd1 = (gb2 − eb) − (g1 − eb) 2 IA, and gd1g2 − eb = gb1(gb2 − gd1 ) 2 IA, and from this it is not difficult to conclude (fgb − eb j g 2 Gg) = IA . Besides the norm we also have a k-linear trace X T : kS −! k; a = a s 7! a(e ) = a ; sb S eS s2S such that T (ab) = T (ba) for each a; b 2 ck(k)S: 1.1.8. Involution. — If S = G is a group, then the ring kG has an involution: ∗ X X −1 : kG −! kG; aggb 7! aggd g2G g2G which is k-linear and satisfies (x∗)∗ = x, (xy)∗ = y∗x∗. Hence any left kG-module M is actually also a right kG-module via mx := x∗m for any m 2 M and x 2 kG. 1.1.9. The center c(kG). — Let us consider the center of the ring kG c(kG) = ckG(kG) = fa 2 kG j ab = ba for any b 2 kGg = ckG(kecG [ Gb) P which clearly contains the commutative unitary ring ck(k). Let a = g2G aggb 2 ckG(kG) then P P a(xecG) = g2G agxgb = (xecG)a = g2G xaggb for each x 2 k implies ag 2 ck(k) for each g 2 G; moreover, for each h 2 G we must have X X X X abh = agghc = agh−1 gb = bha = aghgc = ah−1ggb g2G g2G g2G g2G 3 that is (using the functional notation) (abh)(g) = (bha)(g) for each g; h 2 G , a(gh−1) = a(h−1g) for each g; h 2 G this tourns out to be equivalent to the following condition a(h−1gh) = a(g) for each g; h 2 G: Hence a 2 ckG(kG) if and only if a is a function from G to k with finite support such that: (1) a takes values in ck(k); (2) a(h−1gh) = a(g) for each g; h 2 G, this means that a has to be constant on each conjugacy class of G, that is a is a class function. P Let C ⊆ G be a finite coniugacy class of G, then σC := g2C gb 2 ck(k)G ⊆ kG satisfies both the above requirements and therefore σC 2 c(kG). On the other hand it follows easily that c(kG) is a free ck(k)-module with bases fσC j C is a finite coniugacy class of Gg. 1.2. Examples. — n h ∼ 1.2.1. kCn. — Let Cn = fz 2 C j z = 1g = fζ j h = 0; : : : ; n − 1g =< ζ >= Z=nZ, where 2π i n−1 ζ = e n 2 C, be the cyclic group of order n. Then we have the group ring ZCn = Z⊕Zζb⊕· · ·⊕Zζ[, and the unitary ring homomorphism Z[t] −! ZCn; p(t) 7! p(ζb) (1) ∼ n ∼ ∼ n clearly induces an isomorphism ZCn = Z[t]=(t −1). More generally kCn = k ⊗Z ZCn = k[t]=(t −1) for any unitary ring k. 2 3 4 Note that, for n = 5 we have ab = 1 in ZC5 with a = 1 − ζb − ζb and b = 1 − ζb− ζb , hence U(ZC5) is strictly bigger than U(Z)Cc5 = ±Cc5. ∼ 1.2.2. kC1. — Let C1 =< ζ >= Z be an infinite cyclic group. Then we have the group ring C = L ζn, and the unitary ring homomorphism Z 1 n2Z Zc Z[t] −! ZC1; p(t) 7! p(ζb) ∼ −1 ∼ ∼ −1 clearly induces an isomorphism ZCn = Z[t]t = Z[t; t ]. More generally kC1 = k ⊗Z ZC1 = k[t; t ] for any unitary ring k. 1.2.3. kΣ3. — Let Σ3 be the permutation group of order 6. It can be shown that, for any characteristic zero field K, we have ∼ KΣ3 = K × K × Mat2(K): 1.2.4. kQ. — Let Q be the quaternion group of order 8. It can be shown that ∼ ∼ RQ = R × R × R × R × H; while CQ = C × C × C × C × Mat2(C): 1.3. Linear representations. — Let G be a group. We know that the category of k-linear repre- sentions is equivalent to the category of left kG-modules. Actually, to give a linear representation of G on a k-left module M ρ: G −! Autk(M) is the same as giving a unitary (k-linear) ring homomorphism ': kG −! Endk(M): Hence ring theoretic relations holding in kG are also valid in Endk(M). (1) By definition, if G is finite, ZG is always a free abelian group of rank jGj. If we consider the smallest subring of C 2π i 2π i 2 containing ζ we find Z[ζ] = fp(ζ) 2 C j p(t) 2 Z[t]g.
Details
-
File Typepdf
-
Upload Time-
-
Content LanguagesEnglish
-
Upload UserAnonymous/Not logged-in
-
File Pages9 Page
-
File Size-