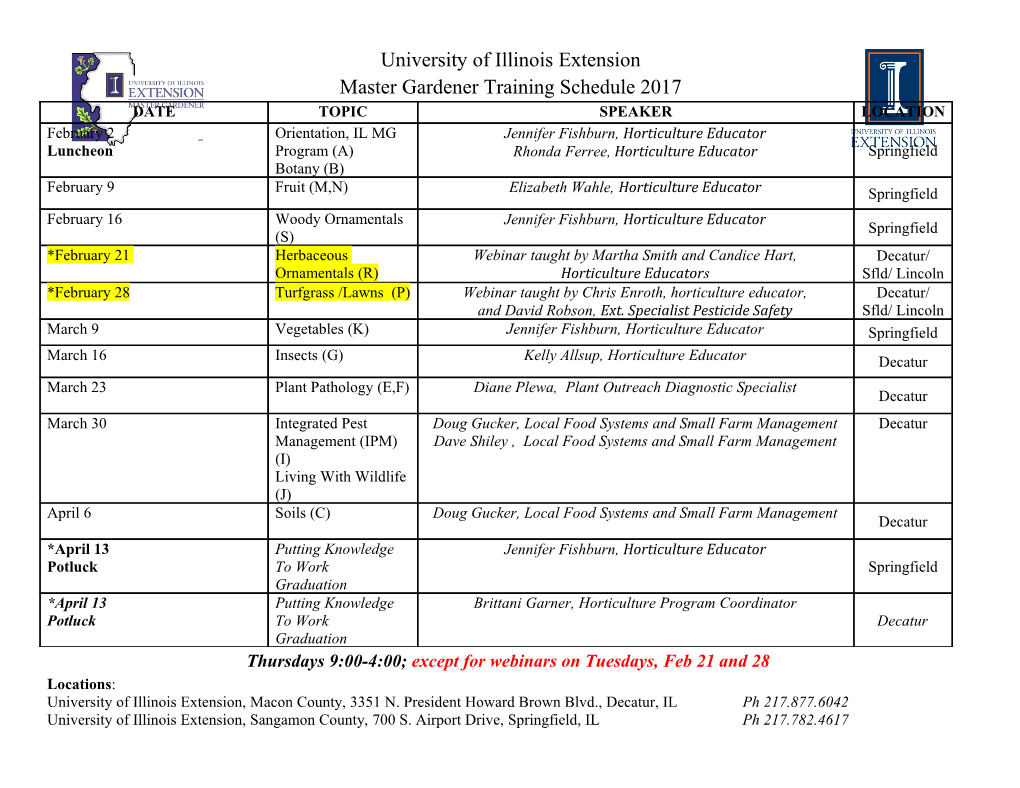
bubble is in resonance, it is well-coupled to the sound field, it can absorb energy efficiently, and it can grow rapidly in a single cycle. Once it has grown, however, Applications it is no longer well-coupled to the sound field. At this point, the surface tension of the liquid combined with the next com- pression wave implosively collapse the of Ultrasound bubble on a submicrosecond time frame. A shock wave can be generated in the gas of the bubble in addition to the simple compressional heating of the gas. to Materials When gas is compressed, heating results. When gas is compressed this rapidly, the heating is nearly adiabatic. The heat has no time to flow out, so a very localized, Chemistry transient hot spot forms, and that hot spot is responsible for the chemistry that Kenneth S. Suslick is observed. The conditions formed during that transient cavitation are extreme. We have been able to measure temperatures and The following is an edited transcription of compresses this cavity, then another ex- pressures by comparative rate ther- the address that Kenneth S. Suslick, recipient pansion wave re-expands it. So we have mometry and by using sonolumines- of an MRS Medal Award, gave at the 1994 an oscillating bubble going back and cence as a spectroscopic probe of the MRS Fall Meeting. Suslick received this forth, say, 20,000 times a second. species formed during cavitation. Our honor for "incisive studies of the chemical As this bubble oscillates, it grows current best estimates of the hot-spot effects of ultrasound on solids and surfaces through several mechanisms, one of conditions give temperatures above and the use of sonochemistry as a new syn- which is rectified diffusion. In rectified 5000 K, pressure of about 1700 atm, and thetic approach to unusual, inorganic com- diffusion, the surface area on expansion time duration under 100 ns, and the time pounds or materials." is slightly larger than on recompression, may be substantially less than that. We so growing processes are kinetically therefore have cooling rates associated 1 This article will begin with an intro- slightly faster than shrinking processes. with this process of more than 10 " de- duction to acoustic cavitation, the physi- This oscillating, growing bubble reaches grees/s. For calibration purposes, if I cal phenomenon responsible for the a resonant size determined by the fre- thrust a poker of red-hot iron into ice chemical effects of ultrasound. Some re- quency of the sound field. When the water, I get a cooling rate of a few thou- cent applications of sonochemistry to the synthesis of nanophase and amorphous metals, as well as to heterogenous cataly- sis, will then be highlighted. Finally, we will examine the effects of ultrasound on Compression metal powders in liquid-solid slurries. Cavitation The chemical effects of ultrasound do not come from a direct interaction of Expansion sound with molecular species. Ultra- sound has frequencies from around 150 -| 15 kilohertz to tens of megahertz. In liq- IMPLOSION uids, this means wavelengths from cen- timeters down to microns, which are not molecular dimensions. Instead, when 100- sound passes through a liquid, the for- SHOCKWAVE mation, growth, and implosive collapse of bubbles can occur, as depicted in Fig- ure 1. This process is called acoustic 50- cavitation. .52 More specifically, sound passing RAPID FORMATION through a liquid consists of expansion CD QUENCHING waves and compression waves. As sound passes through a liquid, if the expansion 100 200 300 400 500 wave is intense enough (that is, if the sound is loud enough), it can pull the liq- Time . sec) uid apart and form a bubble (a cavity). The compression wave comes along and Figure 1. Transient cavitation. MRS BULLETIN/APRIL 1995 29 Applications of Ultrasound to Materials Chemistry scale application of ultrasound that I know for the physical processing of a liquid was for coal benefication at 20 tons/h. Applications The Synthesis of Amorphous and Nanoscale Materials Given the unusual conditions created during cavitation, we considered various potential applications, one of which was the possibility of using ultrasound as a way of generating amorphous and nanoscale materials. Amorphous metals have unusual magnetic, electronic, and catalytic properties. To form an amor- phous metal we need high cooling rates (above a million degrees per second) so that the material can be frozen before it crystallizes. Thermal quenching of molten metal usually requires the addi- tion of nonmetal alloying components; boron is common. Consequently, mak- ing pure amorphous iron has proven Figure 2. Islands of chemistry as a function of time, pressure, and energy. Adapted difficult. from "The Chemical Effects of Ultrasound," by Kenneth S. Suslick. Copyright© We realized that given these cavita- 1989 by Scientific American Inc. All rights reserved. tional hot spots, sonochemistry provides enormous cooling rates fast enough to cause solidification before crystallization sand degrees per second. If I splatter ply (Figure 3). This commercially avail- can occur. However, the primary reac- molten metal onto a liquid-nitrogen- able apparatus can be thermostated and tion site of cavitation is the gas phase in- cooled surface, I get cooling rates of a few the atmosphere above the solution can be side the bubble, in which case, we need a million degrees per second. We will re- controlled. It is useful for small-scale way of producing metal inside the cavita- turn to the implications of these cooling work, which is mostly what we do. Note tion event. Drawing on the same ideas rates later. that large-scale processing of liquids used in organometallic chemical vapor This understanding of cavitation al- with ultrasound also exists. Large-scale deposition (CVD), we need a volatile pre- lows us to compare sonochemistry with cleaning baths are available, and are cursor. We initially considered metal car- other forms of chemistry. Fundamen- used, for example, by the military, to bonyls and metal nitrosyls. When they tally, chemistry is the interaction of en- clean intact jet engines. Flow reactors are irradiated with ultrasound, we are ergy and matter. The parameters that also exist and are commercially available able to generate metals from iron and co- control that interaction are the time of in stackable 20 kW units. The largest balt complexes. From the early transition the interaction, the amount of energy in metals we tend to form metal carbides. the interaction, and the pressure, which We used sonoluminescence as a spec- together describe the three-dimensional troscopic probe to see if, in fact, we can space depicted in Figure 2. This figure strip the ligands off of our precursors. If shows the heavily overpopulated island Power Supply we start with iron pentacarbonyl, and ir- of thermal chemistry at medium pres- radiate with ultrasound in, say, a dode- sure, time, and energy. For high-pres- cane solution, the light coming out is sure, long-time scales such as occur emission from excited-state iron atoms. under geological conditions, the graph This shows that iron pentacarbonyl is be- shows the spiked island of piezochem- ing sonochemically decomposed to iron istry. The island of sonochemistry is atoms, and that some of those iron atoms near photochemistry and flame chemis- Stainless Steel are in electronically excited states. Simi- try. All of these are related because they Collar & O-Rings lar atomic emission is observed from are all forms of interacting energy and other volatile organometallics. matter. However, each has its own Once we have these volatile precursors specific characteristics because each oc- in the cavitation event and we strip off cupies a different region of this three- the ligands, we can form small clusters dimensional space. of metal atoms in that cavitation bubble To introduce ultrasound into solutions and can work with them. As shown in in the laboratory, we use a high-intensity Figure 4, we can let them agglomerate to ultrasonic horn that consists of a solid ti- form amorphous metals. We can trap tanium rod connected to a piezoelectric them with a polymeric ligand, such as ceramic and a 20 kHz, 500 V power sup- Figure 3. Sonochemical apparatus. polyvinylpyrrolidone and form a 30 MRS BULLETIN/APRIL 1995 Applications of Ultrasound to Materials Chemistry add the polymer to the iron pentacar- bonyl solution and irradiate at room tem- perature with high-intensity ultrasound. If we want to make a supported catalyst, we can do the same thing, except we would replace the polymer with an oxide support. We have characterized these extensively. Initially, as formed, the colloids are also amorphous. Electron microdiffraction shows no crystallinity associated with them, and they undergo a one-time irreversible crystallization exotherm in the DSC. These very soft magnetic materials show virtually no hysteresis in magne- tization curves. In magnetic-property studies done in conjunction with Myron Salamon, a professor of physics at UIUC, the nanophase amorphous iron in bulk is a very soft ferromagnet. Its magnetic mo- ment lies between crystalline iron and n = 10- 1000 molten iron. We have agreement in mag- netic measurement from SQUID and neutron-diffraction data. The effective exchange is relatively modest between Figure 4. The sonochemical synthesis of amorphous metals, nanocolloids, and the irons — about 30% of crystalline supported catalysts. iron—and this has been modeled with a correlated spin-glass random packing nanocolloid; or we can deposit these formation of carbidic or oxide phases. model. small clusters on an oxide support, such If we want to trap the material in the The nanocolloidal iron is superpara- as silica, and form supported heteroge- small nanometer-cluster size, we can use magnetic (that is, essentially a single do- nous catalysts. a weakly coordinating ligand, such as main ferromagnet that can be thermally In fact we have been able to do all poly vinylpyrrolidone.
Details
-
File Typepdf
-
Upload Time-
-
Content LanguagesEnglish
-
Upload UserAnonymous/Not logged-in
-
File Pages6 Page
-
File Size-