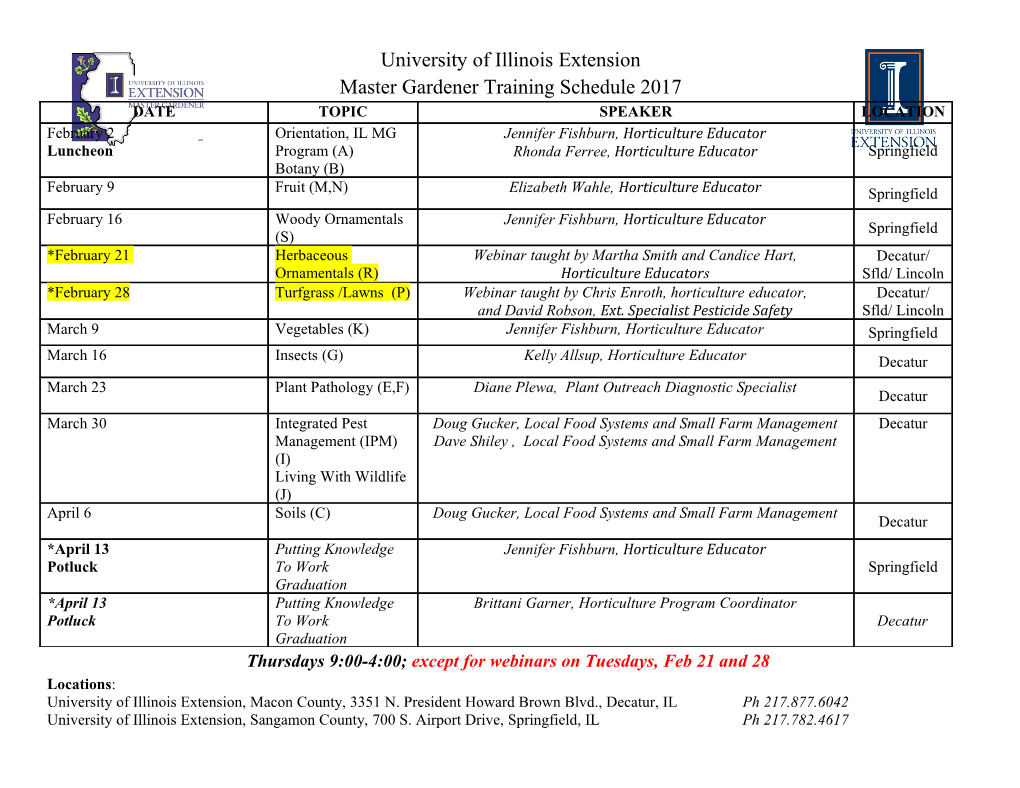
Wetlands (2013) 33:1151–1163 DOI 10.1007/s13157-013-0470-5 ARTICLE Litter Decomposition, and Associated Invertebrate Communities, in Wetland Ponds of the Copper River Delta, Alaska (USA) Scott D. Tiegs & Sally A. Entrekin & Gordon H. Reeves & Deyna Kuntzsch & Richard W. Merritt Received: 26 November 2012 /Accepted: 30 July 2013 /Published online: 21 August 2013 # Society of Wetland Scientists 2013 Abstract High-latitude wetlands provide vital ecological Litter in outwash ponds also had greater invertebrate abun- functions, many of which rely on the decomposition of plant dance than uplift ponds, a pattern driven by collector-gatherer litter, but little understanding exists of how decomposition chironomids. Invertebrate activity was deemed a minor source rates vary across space, and among common plant species. of litter-mass loss relative to microbial decomposition. Results We investigated the litter decomposition of seven plant species suggest that litter-associated invertebrate communities differ in six wetland ponds on the Copper River Delta (Alaska, between pond types, but that differences in plant-litter decom- USA), and the litter-associated invertebrates. The ponds exist position are subtle. on common geomorphic surfaces of the delta: surfaces created by glacial retreat and outwash, and those resulting from Keywords High-latitude wetlands . Plant litter . Leaf-litter uplifted intertidal area following a powerful 1964 earthquake. breakdown . Macroinvertebrates . Wetland functioning An eight-fold range in decomposition rates existed across litter species and correlated with phosphorus (r=0.63), but not nitrogen and carbon content of the litter. Macroinvertebrate Introduction abundance also differed among leaf species. Litter-decay rates did not differ between pond types when expressed on a High-latitude wetlands serve vital ecological functions includ- percent-mass-loss per-day basis; however, on a per-degree- ing climate regulation, nitrogen cycling, and habitat availabil- day basis, decomposition in outwash ponds was more rapid. ity, and many of these hinge on the decomposition of organic matter. For example, given that about a third of global soil Electronic supplementary material The online version of this article carbon is stored in northern peatlands (Gorham 1991), (doi:10.1007/s13157-013-0470-5) contains supplementary material, organic-matter decomposition in high-latitude wetlands is which is available to authorized users. believed to be a significant regulator of atmospheric green- S. D. Tiegs (*) house gases. At more-local scales, organic-matter decompo- Department of Biological Sciences, Oakland University, Rochester, sition plays a role in the successional rates of wetlands, and by MI 48309, USA e-mail: [email protected] extension the long-term viability of habitats such as areas of open water. S. A. Entrekin Plant litter is a dominant source of carbon in most wetlands, Department of Biology, University of Central Arkansas, Conway, and its decomposition is a fundamental ecosystem-level pro- AR 72035, USA cess that is governed by: 1) intrinsic factors related to litter G. H. Reeves quality, and 2) extrinsic factors related to the wetland environ- USDA Forest Service, Pacific Northwest Research Station, Corvallis, ment. For example, litter species (Webster and Benfield 1986), OR 97331, USA the presence of secondary compounds (Gessner and Chauvet D. Kuntzsch 1994; Kraus et al. 2003), and nutrient content of the litter USDA Forest Service, Cordova Ranger District, Cordova, AK (Enríquez et al. 1993) are intrinsic factors that influence litter 99574, USA decomposition in aquatic and terrestrial ecosystems. Examples of extrinsic factors include invertebrate consumption, temper- R. W. Merritt Department of Entomology, Michigan State University, East ature, and in aquatic habitats, dissolved-nutrient concentrations Lansing,, MI 48824, USA (Graca 2001; Davidson and Janssens 2006;Fennessyetal. 1152 Wetlands (2013) 33:1151–1163 2008; Woodward et al. 2012). Ultimately these factors influ- 1995; but see Van Duzor 2011) relative to other wetlands ence the fate of plant-derived carbon, such as whether the litter (Poi de Neiff et al. 2009). Our study was designed to evaluate is consumed by invertebrates, and if wetlands become net intrinsic (e.g., litter quality) and extrinsic drivers (environmen- sources or sinks for carbon (Updegraff et al. 1995). tal conditions, decomposer abundance) of decomposition, and Increasingly, high-latitude wetlands are influenced by environ- the litter-associated invertebrates on the Copper River mental changes stemming from both natural and anthropogen- Delta. Such information is needed and fundamental to ic origins, and understanding how such changes influence understanding nutrient cycling, successional trajectories, organic-matter decomposition is necessary if the carbon bud- ecosystem functioning, invertebrate communities, and the gets and the overall ecology of these wetlands are to be well overall ecology of these vast but little-studied high-latitude understood. wetland ecosystems. The Copper River Delta parallels 75 km of the south- central coast of Alaska (USA) where it supports about 1,000 km2 of wetlands, making this the largest area of coastal Methods wetlands on the Pacific Coast of North America (Fig. 1a). The wetlands here have been broadly classed into physiographic Site Description zones including tidal flats, marshlands, and glacial outwash plains (Thilenius 1995). In aggregate, these diverse wetlands The Copper River Delta (60° 25′ N, 145° 03′ W) forms where constitute significant habitat that supports migratory water- the Copper River deposits sediment into the Gulf of Alaska fowl, a productive commercial salmon fishery, and species of (Fig. 1). The delta supports about 1,000 km 2 of wetlands that conservation concern (Alaska Department of Fish and Game have been broadly classed into physiographic zones including 2012). tidal flats, floodplains, marshlands, and glacial outwash plains The ecology of the Copper River Delta changed abruptly (Thilenius 1995). These marshlands and plains are inter- after the ‘Good Friday’ earthquake of 1964 (Richter magni- spersed by the reticulated channels of the Copper River, small- tude 9.2), one of the most powerful ever recorded. The earth- er rivers and streams that originate from nearby glaciers, and quake uplifted much of the Copper River Delta by 2–3m, sloughs that drain tidewater and the wetlands. Groundwater converting subtidal areas to intertidal, and intertidal areas to inputs, tidal fluctuations, and beaver activity further compli- supertidal, and subsequently, the formation of large areas of cate the hydrology of the Copper River Delta. Together these ‘uplifted’ freshwater marshland (hereafter termed ‘uplifted diverse wetland types are significant habitat that supports the marsh’) where previously there had been none (Thilenius largest gathering of shorebirds in the western hemisphere, a 1990, 1995). Loss of tidal influence on uplifted marsh productive commercial salmon fishery, and several species of areas following the earthquake isolated uplifted marshes conservation concern (Alaska Department of Fish and Game from marine deltaic sediment inputs (Boggs 2000), 2012). suggesting that post-earthquake accretion stems from the accumulation of undecomposed organic matter, rather than Climate marine sediments. Decomposition rates may therefore be particularly important in regulating the future ecology of The climate of the Copper River Delta is cool maritime, but these wetlands. becomes increasingly continental with distance from coast Here we present a study that was designed to provide and proximity to the Copper River (Thilenius 1990). The information on: 1) how the litter of seven common plant growing season spans approximately 110 days from mid- species on the Copper River Delta decomposes, and 2) how May to mid-September (Boggs 2000). Mean monthly temper- decomposition varies among wetland ponds located on the atures range from −3 °C in January to 12 °C in July (at the two most common geomorphic surfaces of the delta: uplifted Cordova airport; Boggs 2000). Precipitation is abundant with marsh that originated after the 1964 earthquake, and glacial an average monthly precipitation of 20 cm (Boggs 2000). outwash plains. While some information exists on the ecolog- ical succession of uplifted wetlands on the Copper River Delta Outwash Plains and Uplifted Marsh (e.g.,Thilenius 1990, 1995), research has yet to target funda- mental ecosystem-level processes such as primary production Outwash plains and uplifted marshes are the dominant land- or organic-matter decomposition, and how they may vary scapes on the Copper River Delta (Boggs 2000), and ponds on between the younger uplifted marsh and other habitats. An each of these surfaces are abundant (Fig. 1b). Outwash plains additional important knowledge gap relates to the relative consist of glacial deposits that are interwoven by the active and contribution of high-latitude wetland invertebrates to the pro- abandoned channels of small streams. Groundwater inputs into cess of decomposition, the communities of which have these systems are common. Uplifted marsh was previously an remained largely unexplored (as identified in Thilenius intertidal area that was uplifted and made super-tidal by the Wetlands (2013) 33:1151–1163 1153 Fig. 1 a Map with inset showing the location of the Copper River delta and approximate locations of the study ponds and b low-elevation aerial photograph showing
Details
-
File Typepdf
-
Upload Time-
-
Content LanguagesEnglish
-
Upload UserAnonymous/Not logged-in
-
File Pages13 Page
-
File Size-