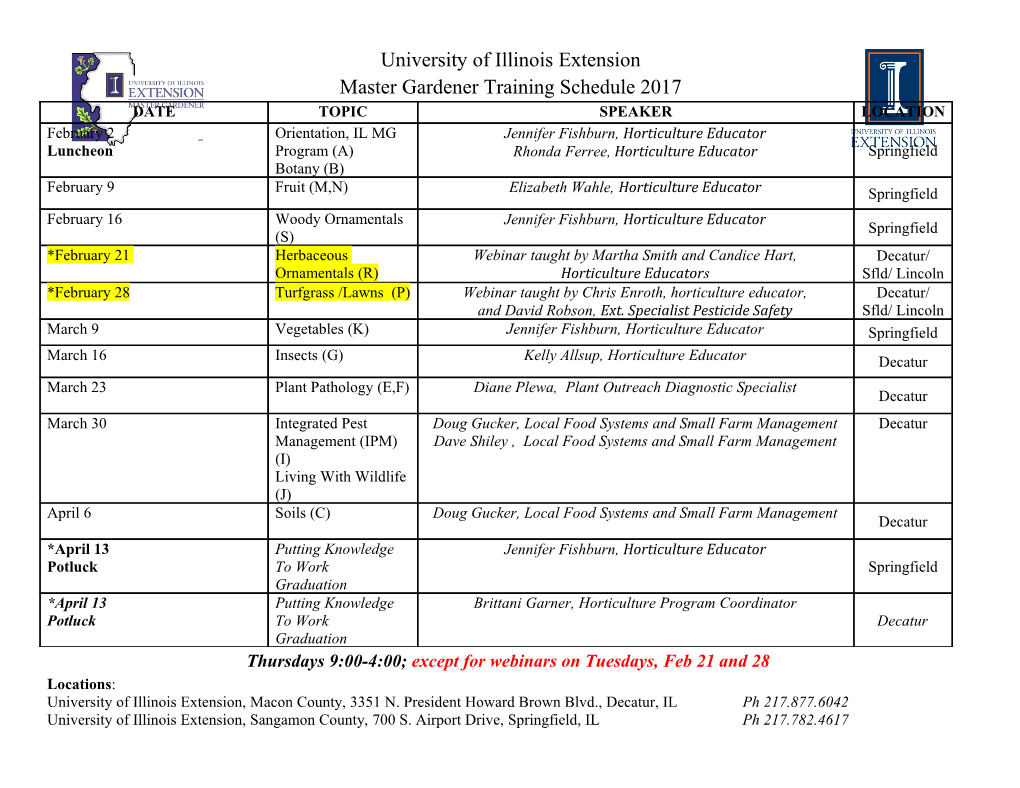
Università degli Studi di Pavia Dipartimento di Biologia e Biotecnologie “L. Spallanzani” Molecular study of the Burkholderia cenocepacia division cell wall operon and FtsZ interactome as targets for new drugs Gabriele Trespidi Dottorato di Ricerca in Genetica, Biologia Molecolare e Cellulare XXXIII Ciclo – A.A. 2017-2020 Università degli Studi di Pavia Dipartimento di Biologia e Biotecnologie “L. Spallanzani” Molecular study of the Burkholderia cenocepacia division cell wall operon and FtsZ interactome as targets for new drugs Gabriele Trespidi Supervised by Prof. Edda De Rossi Dottorato di Ricerca in Genetica, Biologia Molecolare e Cellulare XXXIII Ciclo – A.A. 2017-2020 Cover image: representation of the Escherichia coli division machinery. Created by Ornella Trespidi A tutta la mia famiglia e in particolare a Diletta Table of contents Abstract ..................................................................................................... 1 Abbreviations ............................................................................................ 3 1. Introduction ........................................................................................... 5 1.1 Cystic fibrosis 5 1.1.1 CFTR defects in CF 5 1.1.2 Pathophysiology of CF 7 1.1.3 CFTR modulator therapies 11 1.2 CF-associated lung infections 12 1.2.1 Pseudomonas aeruginosa 13 1.2.2 Staphylococcus aureus 14 1.2.3 Stenotrophomonas maltophilia 15 1.2.4 Achromobacter xylosoxidans 16 1.2.5 Non-tuberculous mycobacteria 17 1.3 The genus Burkholderia 18 1.3.1 Burkholderia cepacia complex 20 1.3.2 Bcc species as CF pathogens 22 1.4 Burkholderia cenocepacia 23 1.4.1 B. cenocepacia epidemiology 23 1.4.2 B. cenocepacia genome 25 1.4.3 B. cenocepacia pathogenicity and virulence factors 26 1.4.3.1 Quorum sensing 29 1.4.3.2 Biofilm 32 1.4.4 B. cenocepacia antibiotic resistance 33 1.4.4.1 Efflux-pumps 34 1.5 Canonical and alternative treatments against B. cenocepacia 36 1.6 Bacterial cell division 38 1.6.1 The division cell wall operon (dcw) 40 1.6.2 MraZ 42 1.7 The 10126109 compound (C109) 44 2. Aims of the work ................................................................................. 47 3. Materials and methods ....................................................................... 49 3.1 Strains and culture conditions 49 3.2 RNA extraction and reverse transcription (RT)-PCR 50 3.3 Analysis of the dcw transcription units by PCR 50 3.4 β-galactosidase activity assay 52 3.5 5’-rapid amplification of cDNA ends (5’-RACE) 53 3.6 Expression and purification of B. cenocepacia MraZ 54 3.7 Electrophoretic mobility shift assay (EMSA) 56 3.8 Characterization of the interactors of the protein FtsZ 57 3.8.1 Bacterial adenylate cyclase two-hybrid system (BACTH) 57 3.8.2 Construction of the recombinant plasmids 57 3.8.3 BACTH complementation assay 59 3.9 Expression and purification of B. cenocepacia SulA 60 3.10 Expression and Purification of B. cenocepacia FtsA 61 3.11 Expression and purification of B. cenocepacia ZipA 62 3.12 Co-sedimentation assay of FtsZ with ZipA or SulA 62 3.13 Co-sedimentation assay of FtsZ with FtsA and vesicles 63 3.14 Biofilm inhibition assay of B. cenocepacia J2315 in three-dimensional (3-D) lung epithelial cell model 63 3.15 Cytotoxicity assessment in 3-D lung epithelial cell model 64 3.16 Metabolic transformation of C109 in CFBE41o- cultures 65 4. Results ................................................................................................ 69 4.1 Characterization of the dcw operon of B. cenocepacia J2315 69 4.1.1 Identification of the dcw cluster in B. cenocepacia genome 69 4.1.2 Study of the transcriptional organization of the dcw operon 69 4.1.3 Characterization of the dcw cluster promoter sequence and transcription start site 70 4.2 Characterization of MraZ, the transcription factor of the dcw cluster 74 4.2.1 Expression and purification of MraZ 74 4.2.2 Electrophoretic mobility shift assay (EMSA) of MraZ 75 4.3 Study of the FtsZ interactors in B. cenocepacia J2315 in vivo and in vitro 77 4.3.1 Complementation analysis of cell division protein using a Bacterial Adenylate Cyclase Two Hybrid (BACTH) assay 77 4.3.2 Expression and purification of SulA 80 4.3.3 SulA inhibits FtsZ polymerization in vitro 82 4.3.4 Expression and purification of FtsA 83 4.3.5 FtsA-FtsZ interaction in vitro 84 4.3.6 Expression and purification of ZipA 85 4.3.7 ZipA-FtsZ interaction in vitro 87 4.4 Biofilm inhibitory activity of TPGS109 against B. cenocepacia J2315 in the 3-D CFBE41o- cell model 88 4.4.2 Cytotoxicity of TPGS109 in the 3-D CFBE41o- cell model 89 4.4.3 Metabolic transformation of C109 in CFBE41o- cultures 91 5. Discussion and future perspectives .................................................. 93 6. References .......................................................................................... 99 Acknowledgements .............................................................................. 125 List of original manuscripts ................................................................. 127 Abstract Abstract Burkholderia cenocepacia has emerged as an important opportunistic pathogen for patients suffering from cystic fibrosis. This bacterium is able to establish aggressive infections in the lungs, colonizing the viscous mucus covering the airways epithelium. Moreover, B. cenocepacia shows extremely high levels of drug resistance, making its eradication almost impossible. Bacterial divisome is considered a valuable pool of druggable targets, and this was confirmed by the characterization of C109, a potent inhibitor of the FtsZ GTPase activity, effective against B. cenocepacia and a broad- spectrum of Gram-positives and -negatives. To find more putative cellular targets, the division mechanism of B. cenocepacia was explored, starting from the characterization of the division cell wall (dcw) operon. The cluster transcriptional organization was assessed by identifying the transcription units and the transcription start site, as well as the promoter and the binding site of MraZ, the dcw transcriptional regulator. Moreover, the FtsZ interactome was dissected in B. cenocepacia, finding significant differences from the most studied microorganisms. Finally, the biofilm inhibitory potential and the cytotoxicity of the C109 nanosuspension were tested in an in vivo- like three-dimensional lung epithelial cell model. This work provides an overview of the B. cenocepacia division pathway, which will be essential for the target-based design of new drugs, and validate the antimicrobial activity of the C109 in a physiologically relevant model. 1 Abbreviations Abbreviations CF Cystic fibrosis CFTR Cystic fibrosis transmembrane conductance regulator Bcc Burkholderia cepacia complex QS Quorum sensing RND Resistance nodulation division family dcw Division cell wall operon C109 Compound 10126109 TPGS D-α-tocopheryl polyethylene glycol 1000 succinate TPGS109 C109 nanocrystals stabilized with TPGS 5’-RACE 5’-rapid amplification of cDNA ends IMAC Immobilized metal-ion affinity chromatography EMSA Electrophoretic mobility shift assay 6-Fam 6-carboxyfluorescein BACTH Bacterial adenylate cyclase two-hybrid system MBP Maltose binding protein 3 1. Introduction 1. Introduction 1.1 Cystic fibrosis Cystic fibrosis (CF) is the most common genetic disease among Caucasians, caused by mutations on both alleles of the gene CFTR (cystic fibrosis transmembrane conductance regulator). This condition leads to the formation of a defective protein in the whole body, affecting multiple organ systems, in particular lungs and pancreas. Historically, CF was first described by Dorothy Andersen in 1938, since until that time CF symptoms had been associated to celiac disease (Andersen, 1938). Since then, the understanding of the disease increased constantly; in 1946 it was recognized to be genetic with an autosomal recessive transmission pattern (Andersen and Hodges, 1946), while, few years later, in 1950s, CF started to be associated with high sweat chloride concentration and lung infections, not only with mucus abnormalities (Di Sant’Agnese et al., 1953). However, the characterization of the chloride transport defect as the main cause of CF and finally the identification of the CFTR gene occurred only in the 1980s (Kerem et al., 1989; Riordan et al., 1989; Rommens et al., 1989). At present, more than 100,000 people worldwide suffer from CF, but thanks to the improvements of symptomatic treatments, the life expectancy in developed countries has increased from few months in 1950s to more than 40 years now (Burgel et al., 2015). The first characterization of the CFTR gene and protein paved the way to the subsequent studies on the molecular pathology of CF, which result essential to develop a cure for this life-shortening disorder. 1.1.1 CFTR defects in CF The CFTR gene is located on the long arm of the chromosome 7 (7q31.2) and consists of 189kb divided in 27 exons encoding a protein of 1480 amino acids (Bear et al., 1992). It is classified as member of the ATP-binding cassette transporter family (ABC), being its activity dependent on the ATP hydrolysis. However, unlike most proteins of this family, which actively transport substrates against their gradient, it uses the chemical energy of the ATP hydrolysis to conduct anions down their electrochemical gradient 5 1. Introduction forming a channel pore. The mature channel is composed of two transmembrane domains forming an anion-selective pore, as well as two cytoplasmic ATP-binding domains and a regulatory (R) domain that allows the
Details
-
File Typepdf
-
Upload Time-
-
Content LanguagesEnglish
-
Upload UserAnonymous/Not logged-in
-
File Pages243 Page
-
File Size-