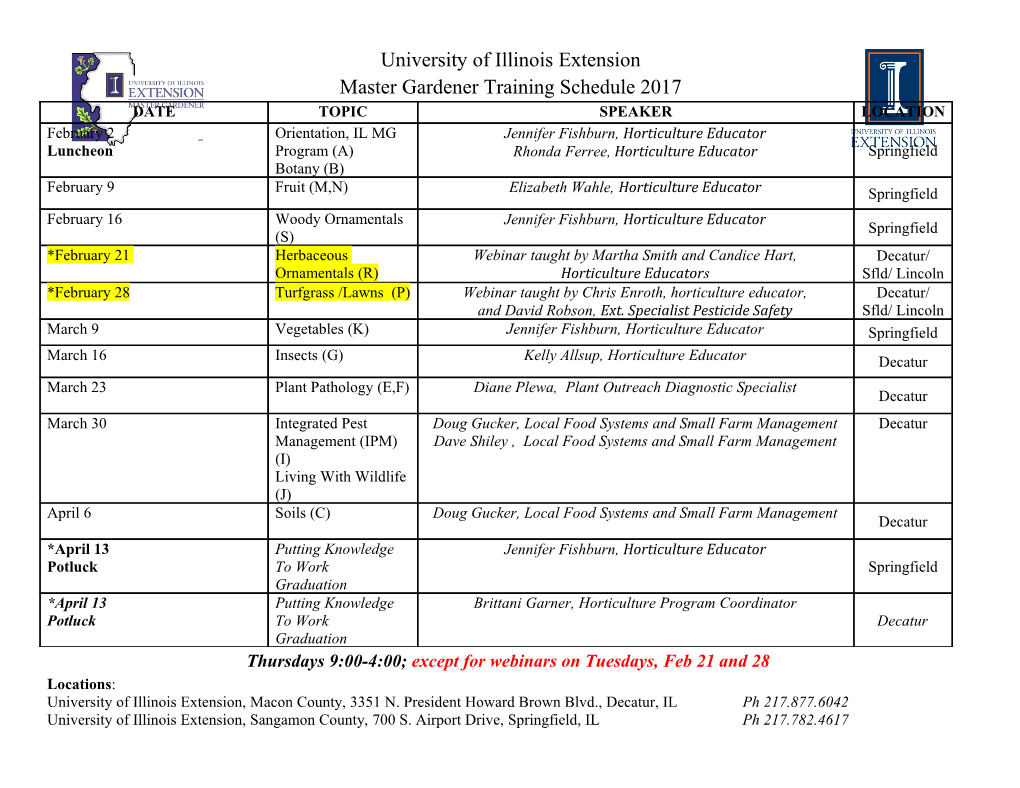
REVIEW ARTICLE https://doi.org/10.1038/s41564-020-0715-z Diversity, ecology and evolution of Archaea Brett J. Baker 1 ✉ , Valerie De Anda1, Kiley W. Seitz1, Nina Dombrowski 1, Alyson E. Santoro2 and Karen G. Lloyd 3 Compared to bacteria, our knowledge of archaeal biology is limited. Historically, microbiologists have mostly relied on cultur- ing and single-gene diversity surveys to understand Archaea in nature. However, only six of the 27 currently proposed archaeal phyla have cultured representatives. Advances in genomic sequencing and computational approaches are revolutionizing our understanding of Archaea. The recovery of genomes belonging to uncultured groups from the environment has resulted in the description of several new phyla, many of which are globally distributed and are among the predominant organisms on the planet. In this Review, we discuss how these genomes, together with long-term enrichment studies and elegant in situ measurements, are providing insights into the metabolic capabilities of the Archaea. We also debate how such studies reveal how important Archaea are in mediating an array of ecological processes, including global carbon and nutrient cycles, and how this increase in archaeal diversity has expanded our view of the tree of life and early archaeal evolution, and has provided new insights into the origin of eukaryotes. nitially, all single-celled, non-eukaryotic microorganisms were 16S rRNA gene sequencing revealed many deeply branching classified as the ‘Prokaryota’1 and, later, the ‘Monera’2. These groups19. This uncultivated diversity is commonly referred to as Iearly classifications lumped Bacteria and Archaea into a single ‘microbial dark matter’. group based primarily on morphology. The development of nucleic In the past ten years, several technological advances in DNA acid-based comparative and phylogenetic analyses by Carl Woese sequencing and computational approaches have enabled the rapid and colleagues provided the first glimpses into the evolutionary reconstruction of genomes from nature, including the descrip- relatedness of microorganisms2,3. These methods showed that life tion of 20 phyla within the Archaea20. Specifically, these advances should be divided into three domains: Eukarya, Eubacteria and include high-throughput sequencing, metagenomic assem- Archaebacteria (with the last two being later renamed Bacteria and bly and binning (that is, the grouping of DNA fragments into Archaea)4–6. A closer examination of Archaea revealed many genetic metagenome-assembled genomes; MAGs), as well as the ability to and biochemical similarities with Eukarya, which led Woese to con- amplify and sequence DNA from a single microbial cell21–25. The troversially propose Archaea to be more closely related to Eukarya accelerated reconstruction of archaeal genomes has led to the dis- than to Bacteria7. Some of the features lacking in Bacteria and shared covery of several new taxonomic groups, in addition to those that among Archaea and Eukarya include the presence of histones, com- had been detected in rRNA diversity surveys. More importantly, plex RNA polymerases and methionine translation initiation8. As the frequent recovery of these lineages illustrates how common, we will review here, the list of similarities between these domains yet unknown, these organisms are. These genomes have enabled is growing with the discovery of new archaeal taxa. Archaea are as a more robust comparison of diversity and evolutionary histories distinct from Bacteria as eukaryotes are; each of the three domains via multi-protein phylogenomics, as well as a more detailed analysis have their own unique genetic, biochemical and cellular character- of the metabolism of uncultivated Archaea (Box 1)26,27. This rate of istics. In fact, Archaea may have more in common with eukaryotes discovery has shown no signs of slowing; in fact, by the time this than Bacteria, such as genomic structure (for example, introns, his- review is published, there will surely be additional phyla added to tones and multiple origins of replication), transcriptional and trans- the tree of life. Looking at the number of available archaeal genomes, lational machinery, and their lack of peptidoglycan in cell walls. it becomes clear that we have a long way to go before we have a com- Particular innovations in DNA amplification, such as up-scaling prehensive sampling of many of the lineages (Fig. 1). There is a large of the polymerase chain reaction (PCR) and lowering the cost of positive bias in the number of genomes that have been obtained sequencing, enabled the generation of large numbers of the 16S from phyla with cultured representatives. Nevertheless, this broader ribosomal RNA (rRNA) gene sequences from the environment. taxonomic sampling of archaeal diversity has allowed the clustering Ribosomal genes are evolutionarily conserved in all known lifeforms of several archaeal phyla into larger taxonomic groups that are now and are therefore ideal marker genes that can be used for phylo- referred to as superphyla (Table 1). Currently, there are three named genetic and taxonomic identification of distantly related organisms. superphyla recognized: Asgard, DPANN and TACK. DPANN and This led to the discovery of a vast, uncultured microbial diver- TACK Archaea were originally named based on the phyla present sity in nature and the realization that our view of microbiology is in them: DPANN referring to the Diapherotrites, Parvarchaeota, often limited by our inability to cultivate microorganisms9–16. Aenigmarchaeota, Nanohaloarchaeota and Nanoarchaeota; and Initially, archaeal rRNA genes fit into two primary phyla, the TACK to the Thaumarchaeota, Aigarchaeota, Crenarchaeota and Euryarchaeota and Crenarchaeota10,17,18. However, broader sampling Korarchaeota28,29 (Table 1). These superphyla now include several of environments began to reveal deep evolutionary branches additional phyla which have been discovered since they were first that did not fall within these two phyla, such as the thermophilic named, which we will detail in this review. At present one phylum, Korarchaeota19. Even within the Crenarchaeota and Euryarchaeota, the Euryarchaeota, does not fall within a superphylum. 1Department of Marine Science, Marine Science Institute, University of Texas Austin, Port Aransas, TX, USA. 2Ecology, Evolution and Marine Biology, University of California, Santa Barbara, CA, USA. 3Microbiology Department, University of Tennessee, Knoxville, TN, USA. ✉e-mail: [email protected] NATURE MICROBIOLOGY | VOL 5 | JULY 2020 | 887–900 | www.nature.com/naturemicrobiology 887 REVIEW ARTICLE NATURE MICROBIOLOGY parti cularly instrumental in advancing our understanding of the Box 1 | Resolving biodiversity and evolutionary histories with biology of this phylum as they have provided numerous cultured phylogenomics representatives for physiological studies and genomic analyses36. Also, the discovery of these Archaea has advanced our understand- Innovations in sequencing technologies and computational ing of the biological adaptations of living in extreme conditions. tools have made it possible to reconstruct large numbers of ge- For example, a species of Methanopyri (Methanopyrus kandleri) nomes from nature. Notably, among these tools are improved has been shown to maintain growth in temperatures up to 122 °C 21,171 metagenomic assemblers and more accurate binning ap- and high pressures of 20 MPa37, which has extended the upper 24,172–175 proaches . Tese approaches result in partial to complete temperature limit of life on the planet. In recent years, in situ geo- genomes, with many of the missing regions explained by dif- chemical and ‘omic’ studies (‘omic’ refers to characterization at culties in assembling short-read genomic data (for example, re- genomic, transcriptomic and proteomic levels) have revealed that peats) rather than signifying truly incomplete genomes. Tis has Euryarchaeota are not just involved in methane production, but led to an explosion of new archaeal genomes and has provided also anaerobic methane oxidation38. Furthermore, it is becoming a framework to build more character-rich and robust phyloge- apparent that these Archaea are also involved in the anaerobic oxi- netic analyses. 16S rRNA gene phylogenies alone have proven dation of other short-chain hydrocarbons39, suggesting that these 176 inadequate in resolving branching patterns among phyla , thus microbes have much more varied roles in biogeochemical cycles multi-loci concatenated trees needed to be employed. It has be- than previously thought. come common to use a reliable subset of ribosomal proteins, and other conserved proteins such as tRNA synthetases, to deline- New branches of Euryarchaeota life. While Archaea are often ate phyla and determine relatedness of phyla within superphy- considered rare members of the microbial biosphere, uncultured 27,88,177,178 la . Tese proteins have been chosen for several reasons: euryarchaeotes have been shown to be dominant members of 179 (1) for their limited lateral gene transfer , (2) for their consist- several ecosystems. For example, Marine Group II and III (MG-II ency in the clustering of phyla with rRNA gene phylogenies and and MG-III; now referred to as Poseidoniales and Pontarchaea) (3) for their broad distribution throughout the tree of life. To Archaea occasionally constitute a large fraction (at times up to date, three phylogenomic clusters of archaeal phyla (superphyla) 40%) of marine microbial communities40–42. Another example have
Details
-
File Typepdf
-
Upload Time-
-
Content LanguagesEnglish
-
Upload UserAnonymous/Not logged-in
-
File Pages14 Page
-
File Size-