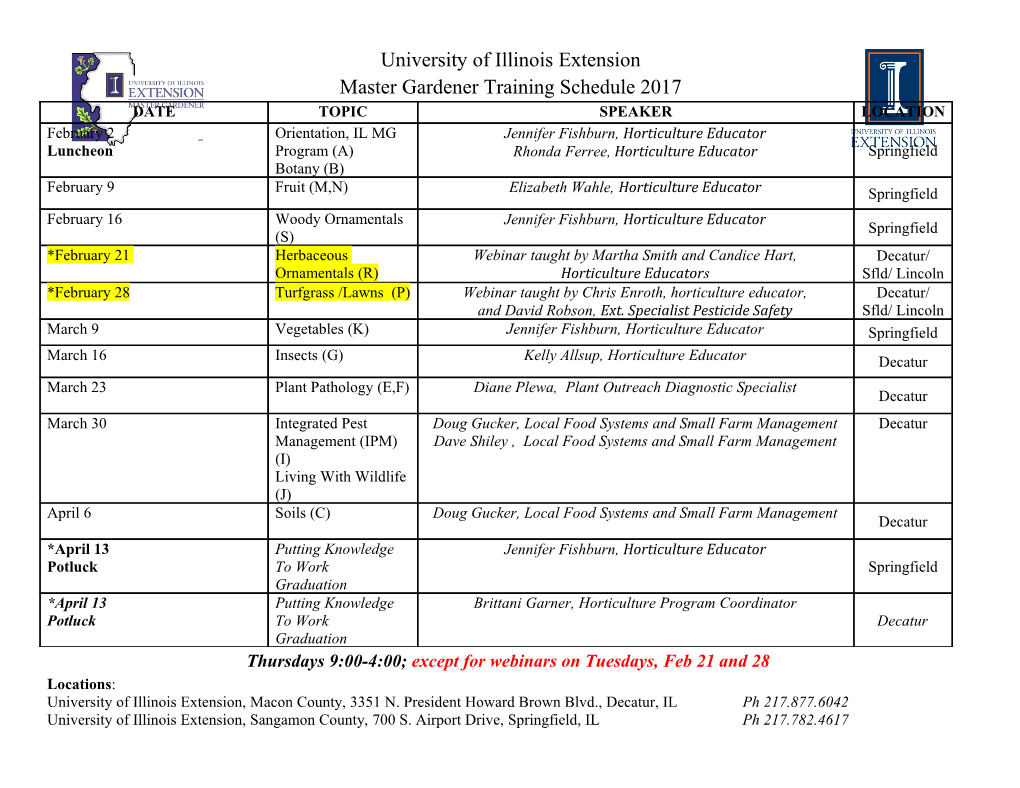
DEMONSTRATION OF REDUCED AIRPORT CONGESTION THROUGH PUSHBACK RATE CONTROL This report is based on the paper submitted to the Ninth USA/EUROPE Air Traffic Management R & D Seminar I. Simaiakis, H. Khadilkar, H. Balakrishnan, T. G. Reynolds and R. J. Hansman Department of Aeronautics and Astronautics Massachusetts Institute of Technology Cambridge, MA, USA B. Reilly Boston Airport Traffic Control Tower Federal Aviation Administration, Boston, MA, USA S. Urlass Office of Environment and Energy Federal Aviation Administration, Washington, DC, USA Report No. ICAT-2011-2 January 2011 MIT International Center for Air Transportation (ICAT) Department of Aeronautics & Astronautics Massachusetts Institute of Technology Cambridge, MA 02139 USA Abstract Airport surface congestion results in significant increases in taxi times, fuel burn and emissions at major airports. This paper presents the field tests of a control strategy to airport congestion control at Boston Logan International Airport. The approach determines a suggested rate to meter pushbacks from the gate, in order to prevent the airport surface from entering congested states and reduce the time that flights spend with engines on while taxiing to the runway. The field trials demonstrated that significant benefits were achievable through such a strategy: during eight four-hour tests conducted during August and September 2010, fuel use was reduced by an estimated 12,000-15,000 kg (3,900-4,900 US gallons), while aircraft gate pushback times were increased by an average of only 4.3 minutes. 1 1 Introduction Aircraft taxiing on the surface contribute significantly to the fuel burn and emissions at airports. The quanti- ties of fuel burned, as well as different pollutants such as Carbon Dioxide, Hydrocarbons, Nitrogen Oxides, Sulfur Oxides and Particulate Matter, are proportional to the taxi times of aircraft, as well as other factors such as the throttle settings, number of engines that are powered, and pilot and airline decisions regarding engine shutdowns during delays. Airport surface congestion at major airports in the United States is responsible for increased taxi-out times, fuel burn and emissions [1]. Similar trends have been noted in Europe, where it is estimated that aircraft spend 10-30% of their flight time taxiing, and that a short/medium range A320 expends as much as 5-10% of its fuel on the ground [2]. Domestic flights in the United States emit about 6 million metric tonnes of CO2, 45,000 tonnes of CO, 8,000 tonnes of NOx, and 4,000 tonnes of HC taxiing out for takeoff; almost half of these emissions are at the 20 most congested airports in the country. The purpose of the Pushback Rate Control Demonstration at Boston Logan International Airport (BOS) was to show that a significant portion of these impacts could be reduced through measures to limit surface congestion. A simple airport congestion control strategy would be a state-dependent pushback policy aimed at re- ducing congestion on the ground. The N-control strategy is one such approach, and was first considered in the Departure Planner project [3]. Several variants of this policy have been studied in prior literature [4, 5, 6, 7]. The policy, as studied in these papers, is effectively a simple threshold heuristic: if the total number of departing aircraft on the ground exceeds a certain threshold, further pushbacks are stopped until the number of aircraft on the ground drops below the threshold. By contrast, the pushback rate control strategy presented in this paper does not stop pushbacks once the surface is in a congested state, instead it regulates the rate at which aircraft pushback from their gates during high departure demand periods so that the airport does not reach undesirably high congested states. 1.1 Motivation: Departure throughput analysis The main motivation for our proposed approach to reduce taxi times is an observation of the performance of the departure throughput of airports. As more aircraft pushback from their gates onto the taxiway system, the throughput of the departure runway initially increases because more aircraft are available in the depar- ture queue, but as this number, denoted as N, exceeds a threshold, the departure runway capacity becomes the limiting factor, and there is no additional increase in throughput. We denote this threshold as N∗. This behavior can be further parameterized by the number of arrivals. The dependence of the departure through- put with the number of aircraft taxiing out and the arrival rate is illustrated for a runway configuration in Figure 1 using 2007 data from FAA’s Aviation System Performance Metrics (ASPM) database. Beyond the threshold N∗, any additional aircraft that pushback simply increase their taxi-out times [8]. The value of N∗ depends on the airport, arrival demand, runway configuration, and meteorological conditions. During periods of high demand, the pushback rate control protocol regulates pushbacks from the gates so that the ∗ number of aircraft taxiing out stays close to a specified value, Nctrl, where Nctrl > N , thereby ensuring that the airport does not reach highly-congested states. While the choice of Nctrl must be large enough to main- tain runway utilization, too large a value will be overly conservative, and result in a loss of benefits from the control strategy. 2 14 13 0 Arrivals /15 min 12 7 Arrivals 11 /15 min 10 14 Arrivals 9 /15 min 8 7 6 5 Takeoff rate (aircraft/15 min) 4 3 2 1 0 0 2 4 6 8 10 12 14 16 18 20 22 24 26 28 Number of aircraft taxiing out Figure 1: Regression of the departure throughput as a function of the number of aircraft taxiing out, param- eterized by the arrival rate for 22L, 27 j 22L, 22R configuration, under VMC [9]. 2 Design of the pushback rate control protocol The main design consideration in developing the pushback rate control protocol was to incorporate effective control techniques into current operational procedures with minimal controller workload and procedural modifications. After discussions with the BOS facility, it was decided that suggesting a rate of pushbacks (to the BOS Gate controller) for each 15-min period was an effective strategy that was amenable to current procedures. The two important parameters that need to be estimated in order to determine a robust control strategy are the N∗ threshold and the departure throughput of the airport for different values of N. These parameters can potentially vary depending on meteorological conditions, runway configuration and arrival demand (as seen in Figure 1), but also on the fleet mix and the data sources we use. 2.1 Runway configurations BOS experiences Visual Meteorological Conditions (VMC) most of the time (over 83% of the time in 2007). It has a complicated runway layout consisting of six runways, five of which intersect with at least one other runway, as shown in Figure 2. As a result, there are numerous possible runway configurations: in 2007, 61 different configurations were reported. The most frequently-used configurations under VMC are 22L, 27 j 22L, 22R; 4L, 4R j 4L, 4R, 9; and 27, 32 j 33L, where the notation ‘R1, R2 j R3, R4’ denotes arrivals on runways R1 and R2, and departures on R3 and R4. The above configurations accounted for about 70% of times under VMC. We note that, of these frequently used configurations, 27, 32 j 33L involves taxiing out aircraft across active runways. Due to construction on taxiway “November” between runways 15L and 22R throughout the 3 09351 BOSTON / GENERAL EDWARD LAWRENCE LOGAN INTL (BOS) AIRPORT DIAGRAM AL-58 (FAA) BOSTON, MASSACHUSETTS ATIS ARR 135.0 US DEP 127.875 CUSTOMS INTERNATIONAL BOSTON TOWER TERMINAL 128.8 257.8 TERMINAL Helicopters 124.725 E GND CON 121.9 TERMINAL CLNC DEL C SATELLITE 121.65 257.8 PIER TERMINAL B 42^23'N CAUTION: BE ALERT TO PIER EMAS RUNWAY CROSSING CONTROL C 190 X 170 TOWER CLEARANCES. READBACK GENERAL MAIN TERMINAL OF ALL RUNWAY HOLDING AVIATION ELEV TERMINAL 22R INSTRUCTIONS IS REQUIRED. RAMP TERMINAL B 15 B N-2 22L N N-3 ELEV N-1 14 R B N VAR 15.5^ W 15R ELEV 215.2^ 15L ELEV 19 M NORTH 15 215.2^ NE-1, 14 JAN 2010 to 11 FEB L CARGO 150.7^ x 150.7^ INTERNATIONAL 2557 X 100 TERMINAL LAHSO A Z N JANUARY 2005 A-1 B 330.7^ ANNUAL RATE OF CHANGE SEE A ELEV M 0.1^ E INSET ELEV N Y 14 20 33R Q 42^22'N Q M ELEV F SATELLITE FIRE 302 16 10083 X 150 STATION F B H ELEV M C 7861 X 150 NE-1, 14 JAN 2010 to 11 FEB 16 A C G A-2 D-2 K C LAHSO D-1 10005 X 150 M D 27 E-2 B E D D E-1 272.1^ ELEV P C K 035.2^ ELEV MAIN FIRE E 14 A 15 STATION K E MM E 14 4L 7000 X 150 B K LAHSO J ELEV ELEV M C 330.7^ 092.1^ 17 17 9 J PAD J (PARKING) J-1 LAHSO 5000 X 100 B M-1 33L ALL AIRCRAFT M HOLD HERE. ELEV EMAS 158 X 170 (4L-APCH) M B 15 035.2^ 71^01'W ELEV 4R 19 42^21'N FIELD ELEV ASDE-X Surveillance System in use. RWYS 4L-22R, 4R-22L, 9-27, 15R-33L, 15L-33R 32 20 Pilots should operate transponders S200, D200, ST175, DT400, DDT800 with Mode C on all twys and rwys. RWY 14-32 S75, D200, ST175, DT400, DDT875 71^00'W AIRPORT DIAGRAM BOSTON, MASSACHUSETTS BOSTON / GENERAL EDWARD LAWRENCE LOGAN INTL (BOS) 09351 Figure 2: BOS airport diagram, showing alignment of runways.
Details
-
File Typepdf
-
Upload Time-
-
Content LanguagesEnglish
-
Upload UserAnonymous/Not logged-in
-
File Pages20 Page
-
File Size-