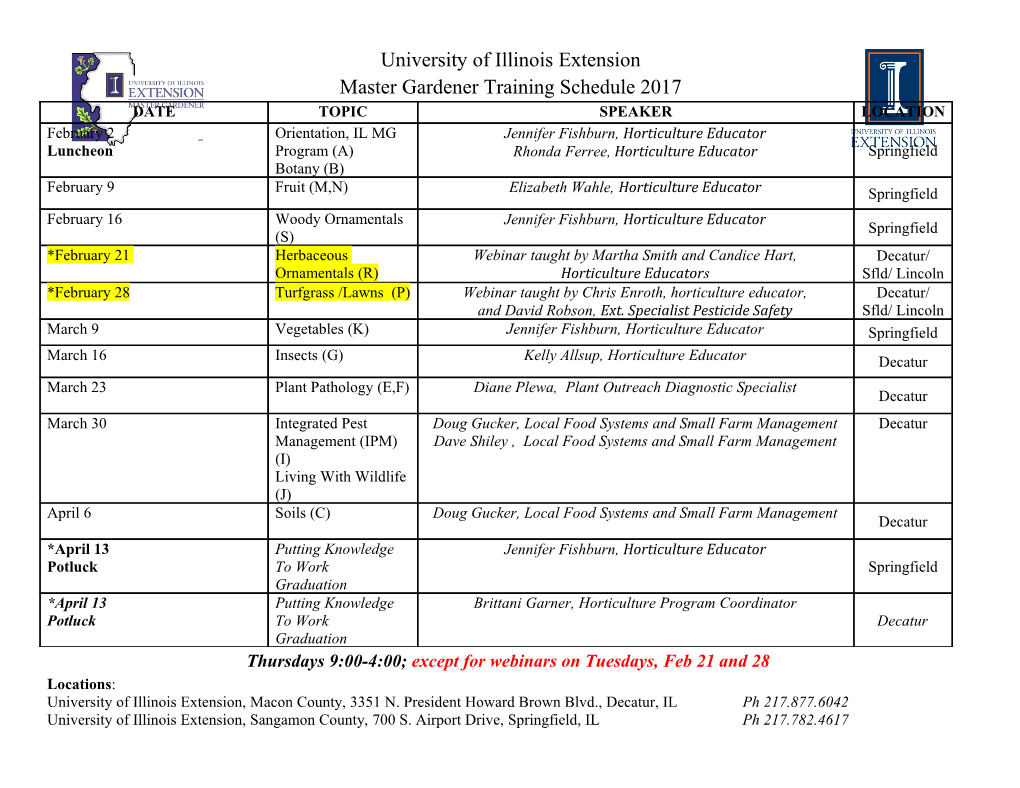
View metadata, citation and similar papers at core.ac.uk brought to you by CORE provided by Elsevier - Publisher Connector Biochimica et Biophysica Acta 1793 (2009) 5–19 Contents lists available at ScienceDirect Biochimica et Biophysica Acta journal homepage: www.elsevier.com/locate/bbamcr Review Cristae formation—linking ultrastructure and function of mitochondria Michael Zick a, Regina Rabl a, Andreas S. Reichert b,⁎ a Adolf-Butenandt-Institut für Physiologische Chemie, Ludwig-Maximilians-Universität München, Butenandtstr. 5, 81377 München, Germany b CEF Makromolekulare Komplexe, Mitochondriale Biologie, Fachbereich Medizin, Goethe-Universität Frankfurt am Main, Theodor-Stern-Kai 7, 60590 Frankfurt am Main, Germany article info abstract Article history: Mitochondria are double-membrane enclosed eukaryotic organelles with a central role in numerous cellular Received 4 March 2008 functions. The ultrastructure of mitochondria varies considerably between tissues, organisms, and the Received in revised form 5 June 2008 physiological state of cells. Alterations and remodeling of inner membrane structures are evident in Accepted 12 June 2008 numerous human disorders and during apoptosis. The inner membrane is composed of two subcompart- Available online 20 June 2008 ments, the cristae membrane and the inner boundary membrane. Recent advances in electron tomography led to the current view that these membrane domains are connected by rather small tubular structures, Keywords: Mitochondria termed crista junctions. They have been proposed to regulate the dynamic distribution of proteins and lipids Cristae formation as well as of soluble metabolites between individual mitochondrial subcompartments. One example is the Crista junction release of cytochrome c upon induction of apoptosis. However, only little is known on the molecular Electron tomography mechanisms mediating the formation and maintenance of cristae and crista junctions. Here we review the Apoptosis current knowledge of the factors that determine cristae morphology and how the latter is linked to Mitochondrial dynamics mitochondrial function. Further, we formulate several theoretical models which could account for the de novo formation of cristae as well as their propagation from existing cristae. © 2008 Elsevier B.V. All rights reserved. 1. Introduction Mitochondria are surrounded by two membranes: the outer membrane (OM) and the inner membrane (IM). Within the inner Mitochondrial research has influenced numerous scientific dis- membrane two distinct regions can be distinguished: the inner ciplines over the last 150 years [1,2]. These organelles have been boundary membrane (IBM) and the cristae membrane (CM). The IBM morphologically described as threads (German, ‘Faden’; Greek ‘mitos’) is adjacent to the outer membrane whereas the CM represents or grains (German, ‘Körper’; Greek ‘chondros’) in various tissues as invaginations of the IBM that protrude into the matrix space. This view early as in the 1850s leading to the name ‘Fadenkörper’ (German) or of a double-membrane enclosed organelle with inner membrane ‘mitochondria’ (Greek). Initially, mitochondrial research was focused convolutions was established with the pioneering work in electron on a number of fundamental metabolic and bioenergetic functions, microscopy of Palade and Sjöstrand in the 1950s [9]. It quickly became such as oxidative phosphorylation (OXPHOS), Krebs cycle, heme clear that mitochondria exhibit an extremely large variability of biosynthesis, β-oxidation of fatty acids, and the metabolism of certain ultrastructures depending on the tissue, the physiological state, and amino acids [2]. The discovery of mitochondrial DNA in the 1960s the developmental stage [10,11]. Tubular, lamellar and even triangle- fueled the scientific discussion about the evolution of mitochondria by shaped cristae membranes (Fig. 1) have been observed [10–12]. endosymbiosis [3]. More recently, targeting and import of proteins Alterations of mitochondrial structures, often accompanied by inclu- into mitochondria [4], biogenesis of Fe/S clusters [5], and the role of sions or aggregates in the mitochondrial matrix, are well known for mitochondria in controlling apoptosis in higher eukaryotes [6] numerous diseases in humans. For example, in lymphoblasts of have substantially extended the interest in mitochondrial research. patients suffering from Barth syndrome mitochondria appear In light of the fundamental roles of mitochondria it is not unexpected enlarged with grossly reduced inner membrane surface area [13]. that dysfunction of this organelle is linked to a wide range of human They mostly exhibit a few but deranged cristae, while also several diseases, including numerous neuropathies and myopathies [7]. giant mitochondria with a completely disorganized inner membrane Furthermore, the accumulation of mutations in mitochondrial DNA topology, in particular concentric stacks of inner membrane or was shown to result in premature ageing of mice [8] providing honeycomb configurations, have been observed. Barth syndrome is a compelling evidence that mitochondrial dysfunction not only pre- mitochondrial disorder caused by mutations in tafazzin [14], which is cedes but also has to be considered as a causative factor in aging of involved in the biosynthesis of mitochondrial phospholipids such as mammals. cardiolipin [15]. This indicates that certain phospholipids are crucial for maintaining mitochondrial ultrastructure. Also in Alzheimer's ⁎ Corresponding author. Tel.: +49 69 6301 87135; fax: +49 69 6301 87162. disease mitochondrial morphology is altered in such a way that E-mail address: [email protected] (A.S. Reichert). cristae are disrupted, and inclusions within mitochondria and varying 0167-4889/$ – see front matter © 2008 Elsevier B.V. All rights reserved. doi:10.1016/j.bbamcr.2008.06.013 6 M. Zick et al. / Biochimica et Biophysica Acta 1793 (2009) 5–19 Fig. 1. Diversity of mitochondrial ultrastructures. Different types of cristae are shown from various tissues under normal (A–D) or pathogenic conditions (E, F): (A) Adrenal cortex. (B) Astrocyte. (C) Fish pseudobranch. (D) Ventricular cardiac muscle (A–D taken from [10]). (E and F). Chronic polymyositis with Raynaud's syndrome (taken from [30]). types of inner membrane arrangements including concentric and 2. Mitochondrial architecture — changing views parallel stacks of cristae membranes were observed [16]. Inclusions and altered cristae morphology were also found in the skeletal muscle The use of electron microscopy in the 1950s boosted mitochondrial of patients with amyotrophic lateral sclerosis [17]. Several other research considerably as it allowed novel insights into the internal examples of human diseases with alterations of mitochondrial structure of mitochondria. However, determining and interpreting morphology have been reported (see Table 1), such as Parkinson's ultrastructural details of mitochondria is a challenging task. On the disease [18–22], Wolf–Hirschhorn syndrome [23–25], Wilson disease one hand it is desirable to obtain high resolution of structural details. [26–28], 3-methylglutaconic aciduria [29], chronic polymyositis [30], On the other hand mitochondria are rather large, elongated tubular and hereditary mitochondrial hypertrophic cardiomyopathy [31]. structures within the cytosol covering the length of up to several Here, we aim to review our current understanding on how hundred µm making the simultaneous analysis of larger volumes mitochondrial function and structure are linked to each other and, difficult. For the elucidation of small membrane structures the use of particularly, what molecular mechanisms are known to mediate electron microscopy is the method of choice. However, sections used cristae formation. for electron microscopy have a certain thickness of at least 5 but M. Zick et al. / Biochimica et Biophysica Acta 1793 (2009) 5–19 7 Table 1 Candidate proteins determining cristae morphology Protein (organism) Aberration of mitochondrial Assigned function Associated human disorder References ultrastructure Atp21p/Tim11p (su e), Onion-like, elongated cristae Dimerization and higher order – [4,84,85,87–89] Atp20p (su g), Atp4p oligomerization components of the (S. cerevisiae) F1FO-ATP synthase ATP6/Atp6p (H. sapiens, Aberrant mitochondrial morphology; Proton channel of F1FO-ATP synthase Neuropathy, ataxia and [95,98,100] D. melanogaster, IM vesicles, septa retinitis pigmentosa (NARP), S. cerevisiae) maternally inherited Leigh syndrome (MILS) PINK1 (H. sapiens, C. elegans, Swollen mitochondria; cristae Mitochondrial serine/threonine kinase "; Parkinson's disease [18,174,178] D. melanogaster) fragmentation Tafazzin (H. sapiens) Fragmentation of mitochondria; Acyltransferase involved in the Barth syndrome (BTHS) [13,73] reduced cristae surface biosynthesis of mitochondrial phospholipids DICE1/DIC-1 (H. sapiens, Aberrant IM ultrastructure, Tumor suppressor protein Various types of cancer [18, 153–155] C. elegans) IM vesiculation (e.g. lung carcinoma, prostate cancer) AIF (M. musculus) Aberrant IM topology Induction of apoptosis – [152] Mdm33p (S. cerevisiae) Aberrant mitochondria; overexpression Fission of inner mitochondrial membrane – [163] leads to loss of cristae Mmm1p (S. cerevisiae) Spherical mitochondria; disorganization Maintenance of mtDNA; import and – [164,165,169] of IM assembly of beta-barrel proteins LETM1/Mdm38p (H. sapiens, Osmotic swelling; loss of membrane K+/H+ exchange; chaperone for Wolf–Hirschhorn syndrome [24,170,171] D. melanogaster,
Details
-
File Typepdf
-
Upload Time-
-
Content LanguagesEnglish
-
Upload UserAnonymous/Not logged-in
-
File Pages15 Page
-
File Size-