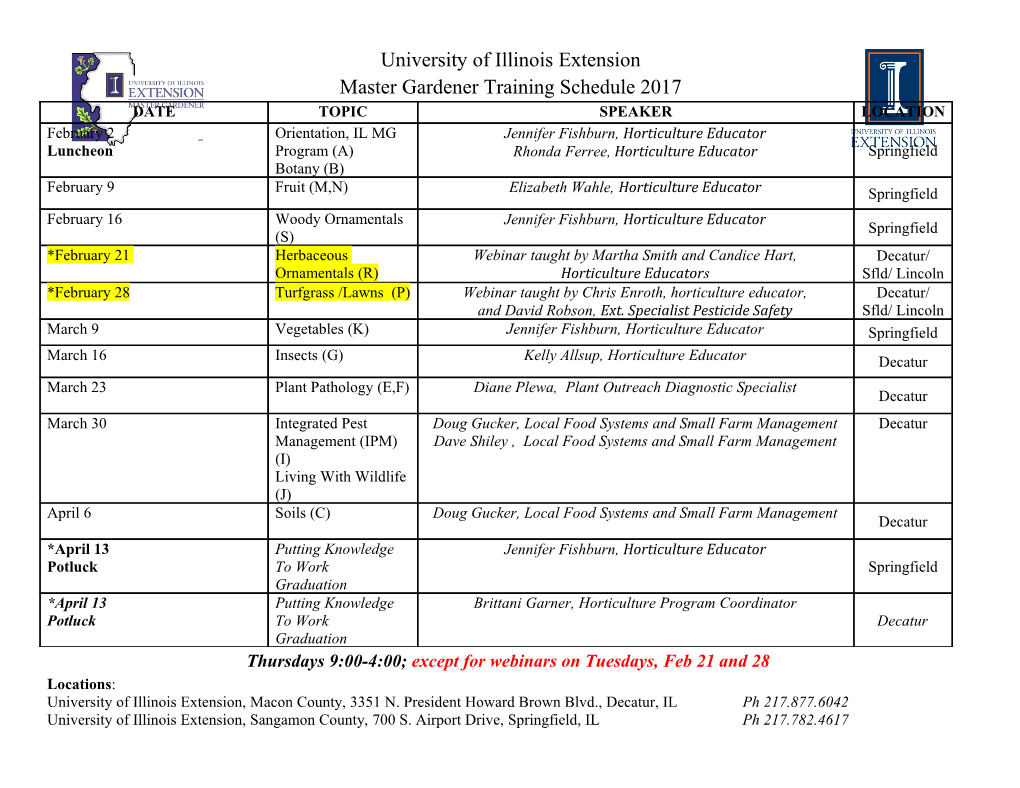
TET enzymes, DNA demethylation and pluripotency Samuel E Ross1, 2 and Ozren Bogdanovic1, 3 1 Genomics and Epigenetics Division, Garvan Institute of Medical Research, Sydney, New South Wales, 2010, Australia 2 St Vincent's Clinical School, Faculty of Medicine, University of New South Wales, Sydney, New South Wales, 2010, Australia. 3 School of Biotechnology and Biomolecular Sciences, University of New South Wales, Sydney, New South Wales, 2052, Australia. Correspondence to: [email protected] Abstract Ten-eleven translocation (TET) methylcytosine dioxygenases (TET1, TET2, TET3) actively cause demethylation of 5-methylcytosine (5mC) and produce and safeguard hypomethylation at key regulatory regions across the genome. This 5mC erasure is particularly important in pluripotent embryonic stem cells (ESCs) as they need to maintain self-renewal capabilities while retaining the potential to generate different cell types with diverse 5mC patterns. In this Review, we discuss the multiple roles of TET proteins in mouse ESCs, and other vertebrate model systems, with a particular focus on TET functions in pluripotency, differentiation, and developmental DNA methylome reprogramming. Furthermore, we elaborate on the recently described non-catalytic roles of TET proteins in diverse biological contexts. Overall, TET proteins are multifunctional regulators that through both their catalytic and non-catalytic roles carry out myriad functions linked to early developmental processes. Introduction Ten-eleven translocation (TET) methylcytosine dioxygenases were first described when TET1 was identified as a fusion partner of the mixed lineage leukaemia gene (MLL) in acute myeloid leukaemia [1]. Since then TET proteins have been associated with other myeloid and lymphoid malignancies as well as solid cancers including melanoma, breast, and prostate cancers [2]. TET proteins play key roles in the regulation of self-renewal capacities of diverse stem cell types, and mutations in genes coding for TET proteins can lead to oncogenic transformation [3]. The major catalytic function of TET proteins was first described in a landmark study, which revealed that TET1 could catalyse the conversion of 5-methylcytosine (5mC) to 5- hydroxymethylcytosine (5hmC) [4]. It is now known that TET proteins can cause the sequential oxidation of 5mC to 5hmC, 5-formylcytosine (5fC), and 5-carboxylcytosine (5caC) [5, 6]. The oxidised intermediates, 5fC and 5caC, can be removed by thymine DNA glycosylases (TDG) and base-excision repair (BER) machinery to regenerate unmethylated cytosines at targeted sites [7, 8]. Specific reader proteins have been described for each of these oxidised intermediates, which is suggestive of their potential function in gene regulation [9]. This ability of targeted 5mC removal associated with TET function is particularly important in embryonic stem cells (ESCs) as they need to maintain self-renewal capabilities as well as adopt diverse 5mC patterns upon differentiation. Mammals and vertebrates such as zebrafish encode three TET protein copies (TET1, TET2, TET3) [4, 10] whereas in the Xenopus genus only TET2 and TET3 have been described [11]. Invertebrate chordates such as amphioxus (Branchiostoma lanceolatum) display a single TET orthologue of yet uncharacterised function [12]. TET proteins are characterised by their core catalytic domain and can exist in cell-type specific isoforms with or without their CXXC binding domain (present in TET1 and TET3, and supplemented in TET2 by its association with CXXC4/IDAX) [13, 14] (Figure 1). In this Review, we focus on insights related to TET protein function in mouse embryonic stem cells (mESCs) and vertebrate embryos. More extensive reviews of TET function have recently been published elsewhere [2, 15]. TET expression and binding dynamics in mESCs ESCs are derived from epiblasts of mammalian blastocysts and cultured in conditions that promote propagation of the pluripotent state. ESCs are considered to be either ‘naïve’ when isolated from pre-implantation epiblasts (E3.5-E4.5), or ‘primed’ when obtained from post- implantation epiblasts (E5.5-E6.5) [16, 17]. Naïve mouse ESCs are generally cultured in two types of media. The original culture conditions consisted of leukaemia inhibitory factor (LIF) and foetal calf serum. While these conditions efficiently maintained the potential for blastocyst chimera formation, cells in these cultures displayed heterogeneous expression patterns of pluripotency genes. Furthermore, such cells exhibited 5mC patterns similar to post- implantation embryos and somatic cells [17]. More recently, culture conditions consisting of serum-free media and two small molecular inhibitors (2i) that block FGF/ERK pathway and partly inhibit glycogen synthase 3 (Gsk3), have been developed [18]. Cells grown in 2i conditions are homogeneous in terms of pluripotency marker expression and morphology, in addition to displaying low 5mC levels characteristic of pre-implantation embryos [19-21]. Such cells are thus naïve both in terms of their cellular potency and their epigenome, and are often referred to as “ground state” naïve ESCs. The majority of earlier studies (e.g. before 2010) routinely used serum/LIF conditions for mESC culture, thus unless otherwise stated, the studies discussed below refer to serum/LIF-cultured mESCs. In mESCs, Tet1 and Tet2 constitute the majority of Tet transcripts while Tet3 is barely detectable [5, 22, 23] (Figure 2). When LIF is removed from the mESC culture media, mESCs spontaneously differentiate and this is marked by a significant decrease in Tet1 and 5hmC levels [4, 22]. In line with these findings, Tet1 levels displayed a progressive drop during the eight days of embryoid body (EB) culture [22]. This is in contrast to Tet2 transcripts that decrease during first four days but are fully restored by day eight, and Tet3 transcripts that displayed a progressive and significant (> 20 fold) increase during this process [22] (Figure 2). It is thus likely that the relatively high 5hmC content (~ 4%) observed in undifferentiated ESCs [4] is predominantly caused by high levels of TET1, and to a lesser extent, TET2. In agreement with these findings, in 2i-cultured ESCs, Tet1 is the most highly expressed Tet transcript whereas Tet3 is undetectable [19]. Interestingly, 2i and serum/LIF conditions differ in the levels of Tet2, which appears to be more highly expressed in 2i [19]. During serum to 2i reprogramming, TET1 and TET2 are required for the production of 5hmC even though they are not the major determinants of the profound 5mC loss associated with this process [19, 20]. Both serum to 2i reprogramming as well as the reprogramming of epiblast stem cells (EpiSCs) to naïve pluripotency can be enhanced by the addition of retinol and ascorbate to the growth medium [24]. These components augment TET activity by different mechanisms; ascorbate directly stimulates TET activity by the reduction of non-enzyme bound Fe3+ to Fe2+, whereas retinol and retinoid acid (RA) increase expression of Tet2 and Tet3 through binding to conserved RA-responsive elements [24]. Genome wide profiling of 5hmC and TET1 protein binding sites have revealed an enrichment of TET1 at CpG island promoters and gene bodies in mESCs, in agreement with the presence of the CXXC domain in TET1 [25-27] (Figure 1). Nevertheless, the exact role of CXXC in TET1 targeting to chromatin is unclear, and it appears that TET1 largely depends on transcription factors such as NANOG, PRDM14, TEX10, and others for its chromatin recruitment [28-30]. TET1 also exhibits strong enrichment at bivalent promoters that are marked by both active (H3K4me3) and repressive (H3K27me3) histone marks [27, 31]. These regulatory signatures frequently decorate promoters of developmental and pluripotency genes [32]. Similarly to TET1, TET2 can also associate with gene bodies [33] and distal regulatory elements such as enhancers [34]. The exact roles of TET protein function in mESCs are far from being fully understood. So far, TETs have been attributed both activator [25] and repressor [26] function, and have been associated with the regulation of bivalent chromatin [31] and Polycomb-marked regions [27]. These functions, when impaired, are believed to impact diverse features of the pluripotent transcriptome with profound consequences for differentiation processes [23, 34] (Table 1). TET proteins play key roles during mESC differentiation Despite the coordinated expression of TETs during pluripotency, TET triple knockout (TKO) mESCs maintained self-renewal capabilities, normal ESC morphology, and expressed pluripotency markers such as Oct4 and Nanog [35, 36]. However, embryoid bodies (EBs) formed from TKO mESCs displayed reduced levels of endo- and mesodermal markers and resulted in poorly differentiated tissues in general [35]. Moreover, TKO teratomas lacked mesodermal and endodermal structures whereas TKO mESCs were characterised by low efficiency of chimeric embryo formation [35]. A number of studies have explored the effects of single and double TET knockouts (DKO) to reveal specialised roles of TET proteins during ESC differentiation (Table 1). TET1 targeting studies have reproducibly found that TET1 depletion in mESCs results in a skew towards extraembryonic, mesodermal or endodermal lineages in embryoid body differentiation assays and teratoma formation assays [5, 23, 25, 36- 38] (Figure 3A). This can be partly explained by the observation that TET1 associates with the transcriptional repressor and activator SIN3A to activate
Details
-
File Typepdf
-
Upload Time-
-
Content LanguagesEnglish
-
Upload UserAnonymous/Not logged-in
-
File Pages21 Page
-
File Size-