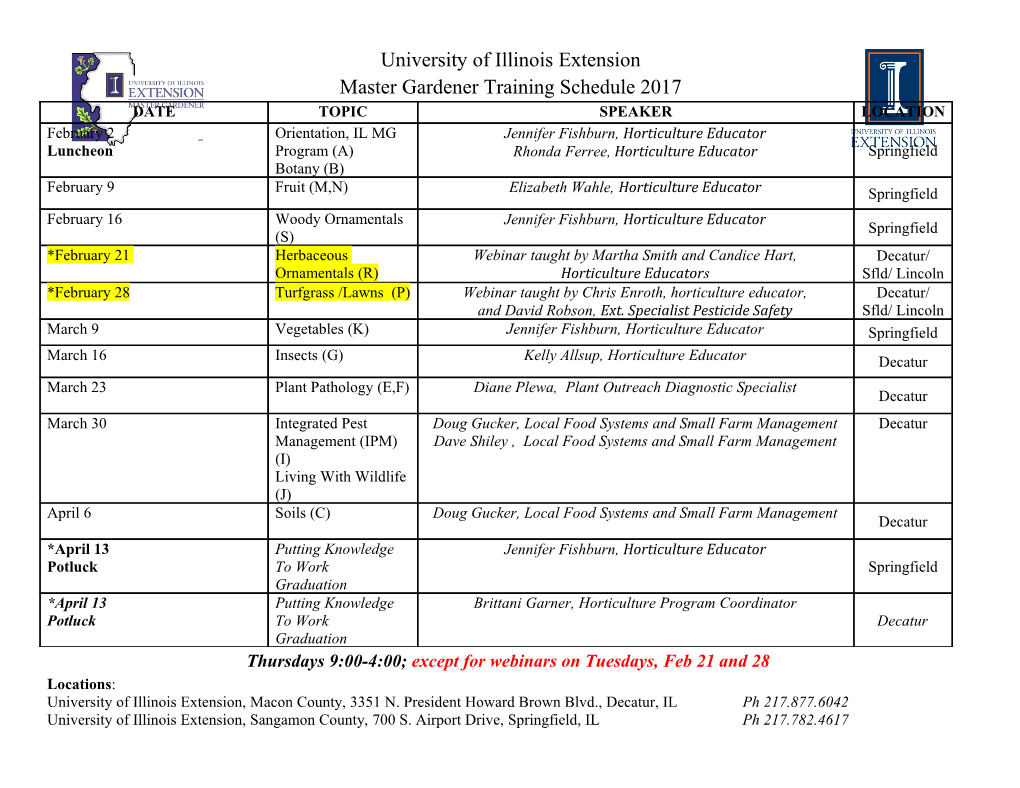
contents Principles of Biology 24 Biological Energy Transfer Cellular respiration involves the stepwise transfer of energy and electrons. Large-scale municipal composting. The steam emitted from the compost as it is being turned is evidence of trillions of microorganisms performing cellular respiration, breaking down molecules in the compost and generating energy, some in the form of heat. Nancy J. Pierce/Science Source. Topics Covered in this Module How Do Organisms Obtain Energy? Redox Reactions An Outline of the Stages in Cellular Respiration Major Objectives of this Module Describe the relationships among photosynthesis, respiration, producers, and consumers. Explain how reduction-oxidation (redox) reactions work. Describe how aerobic cellular respiration breaks down fuel molecules and releases energy for cellular work. page 121 of 989 4 pages left in this module contents Principles of Biology 24 Biological Energy Transfer How Do Organisms Obtain Energy? No matter how wealthy you become in life, you will always work for a living. That is, your cells will always be working to keep you alive. From synthesizing proteins to producing gametes to chasing down prey, living cells are constantly at work, and all that work requires energy. Where does that energy come from? Ultimately it comes from our Sun as light energy. Energy flows through all living systems. Plants, algae, and photosynthetic bacteria use energy from sunlight to generate sugar molecules through the process of photosynthesis. Such organisms, known as photoautotrophic producers, convert the radiant energy of sunlight into chemical energy that they store in sugars and other organic compounds. Other organisms, termed heterotrophic consumers, must acquire the chemical energy they need by ingesting or absorbing organic molecules from other organisms. In both autotrophs and heterotrophs, cellular respiration is the process that releases energy through the breakdown of these food sources. This energy is then used to fuel cellular processes. A common misconception is that producers, such as plants, only conduct photosynthesis and need not perform cellular respiration. However, producers have to conduct respiration to break down the organic molecules they have produced (Figure 1), just as consumers do in order to release chemical energy from the food they consume. Carbohydrates, fats, and proteins can all be metabolized via cellular respiration. Each of these large molecules is broken down into its smaller components, namely, sugars, smaller fats, and amino acids. These smaller molecules are fed into the cellular respiration process, where chemical energy is generated. Fats are particularly generous energy producers. A gram of fat yields more than twice as much ATP as a gram of carbohydrate. A gram of fat has 9 kcal or 38 kJ. (Nutritional calories, which are the values reported on food packages, are actually kilocalories or kcal.) Carbohydrates and protein each have 4 kcal per gram. Figure 1: Overview of photosynthesis and aerobic respiration. Photosynthetic organisms — plants, algae, and some bacteria — use light energy to generate organic molecules from carbon dioxide and water. All organisms, including photosynthetic organisms, perform some form of cellular respiration to break down organic molecules. In aerobic respiration, molecular oxygen is consumed and water and carbon dioxide are produced. Some of the energy released from the organic molecules is temporarily stored in ATP. The exergonic hydrolysis of ATP can then be coupled to endergonic reactions; some energy is always released as heat. © 2013 Nature Education All rights reserved. Energy flows into an ecosystem from the Sun and moves from producers to consumers through a food web. We can follow the energy flow through an ecosystem a bit more closely using an energy pyramid diagram, which shows how much energy is present at different levels within a food web (Figure 2). Photosynthesizers (the plants in Figure 2) capture only a small fraction of the energy from the solar radiation that strikes the Earth (usually less than 1%), which they convert into stored chemical energy. Once producers convert radiant energy into chemical energy, this energy becomes available to consumers. At the next level, primary consumers are herbivores, which can range in size from tiny worms and insects to massive elephants and bison. Although each species converts consumed plant energy into useful energy at a particular efficiency, 10% efficiency of transfer is a typical rule of thumb. Likewise, only a small proportion of the energy tied up in herbivores makes it up to the level of secondary consumers, which typically feed on herbivores but are themselves often prey to larger, tertiary, or top level, consumers. In all, only the tiniest fraction of energy that enters a given ecosystem through photosynthesis at the base makes it up to these top consumers (Figure 2). Figure 2: An idealized pyramid of energy flow from the Sun into a hypothetical terrestrial food web. Typically, less than 1% of the solar radiation that is available to primary producers is converted into stored chemical energy through photosynthesis. After producers store chemical energy (given as 100% because it can vary among ecosystems), a rule of thumb is that consumers can convert ~10% of the energy from lower trophic levels to their own stored energy (with the rest being lost to metabolism, waste, and other processes). Thus, primary consumers (herbivores) contain ~10% of the energy that was present in the producers they consumed; secondary consumers (predators) have 1% of the energy that was present in the consumed producers; and tertiary consumers have 0.1% of the energy that was present in the consumed producers. Food webs generally end at tertiary consumers because of the exponential loss of energy at each higher level. © 2014 Nature Education All rights reserved. Test Yourself Calculate how many kilocalories of energy a tertiary consumer receives from a producer that takes in 120 kilocalories. Assume for your calculations that 10% of the energy at each level of the food chain is passed on. Submit Catabolic processes break down fuel and release energy. So just how does the oatmeal you ate for breakfast become the energy you use to do work after class? Organic molecules in food, such as carbohydrates, fats and proteins, contain potential energy in the chemical bonds between their atoms. Catabolic processes in the cell decompose large organic molecules into simpler products, releasing the energy held in their chemical bonds. Most of this energy is transferred to and stored in the chemical bonds of adenosine triphosphate (ATP), the molecule that provides the energy to drive the majority of metabolic processes in living organisms. The 6-carbon sugar glucose (C6H12O6) is the most important fuel for cellular respiration. The complete breakdown of glucose during aerobic cellular respiration generates a large amount of ATP in a process that requires oxygen to function. In some organisms, organic molecules can be catabolized without using oxygen. One such anaerobic process is fermentation, in which sugars are partially broken down to produce ATP. Other organisms use anaerobic respiration, in which sulfate or nitrate serve the role that oxygen does in cellular respiration. The overall catabolism of glucose in aerobic cellular respiration can be represented by the following chemical equation: C6H12O6 + 6 O2 → 6 CO2 + 6 H2O ∆G = -686 kcal/mol (-2870 kJ/mol) The negative free energy change indicates that the reaction releases energy. However, if cellular respiration took place in a single step and all of the energy was released at once, it would be a massive release of heat that would likely destroy the cell. To avoid self-destructing and to capture this energy, the cell breaks down glucose over many steps. In this manner, the release of energy is controlled — more is captured and converted to ATP, and less is lost as heat. Redox Reactions During the cellular respiration reactions, what happens that enables the energy of bond breaking to be captured and converted into the formation of ATP? Simply put, a series of chemical reactions moves electrons between molecules. The transfer of electrons releases stored energy that can be used to attach inorganic phosphate to ADP to form ATP. These electron- transferring chemical reactions are called reduction-oxidation reactions, or redox reactions for short. In a redox reaction, two simultaneous reactions are occurring. One reactant donates electrons to another reactant. The electron donor becomes oxidized as it loses electrons. The other reactant, the electron acceptor, becomes reduced as it gains the electrons. Redox reactions are very common in chemistry. For example, one type of redox reaction occurs when elemental magnesium (Mg) and molecular oxygen (O2) combine to form magnesium oxide (MgO). In this reaction, two magnesium atoms donate four electrons to the oxygen molecule (O2). As a result, the magnesium atoms are oxidized into Mg2+ ions, each with a 2+ charge. On the other hand, the oxygen molecule is reduced upon accepting the four electrons, resulting in two O2- ions. The oxidized Mg2+ ions and reduced O2- ions combine to form magnesium oxide (Figure 3). Figure 3: Redox reaction of magnesium and oxygen. A reactant that donates electrons is oxidized, which results in a positive charge. The reactant that accepts the electrons is reduced, which results in a negative charge. © 2013 Nature Education All rights reserved. Test Yourself Determine which atom is oxidized and which is reduced in the following reaction: 2 Al + 3 Br2 → Al2Br6 Submit Electronegativity and redox reactions. What drives electron movement during redox reactions? Electrons tend to be found at different distances from nuclei in different atoms. Because negatively charged electrons are attracted to positively charged nuclei, separating electrons from nuclei requires an input of energy. As a result, the potential energy of the electron increases with distance from the proton. Likewise, allowing an electron to move closer to a nucleus releases some of its potential energy, which can be used to drive other chemical reactions.
Details
-
File Typepdf
-
Upload Time-
-
Content LanguagesEnglish
-
Upload UserAnonymous/Not logged-in
-
File Pages16 Page
-
File Size-