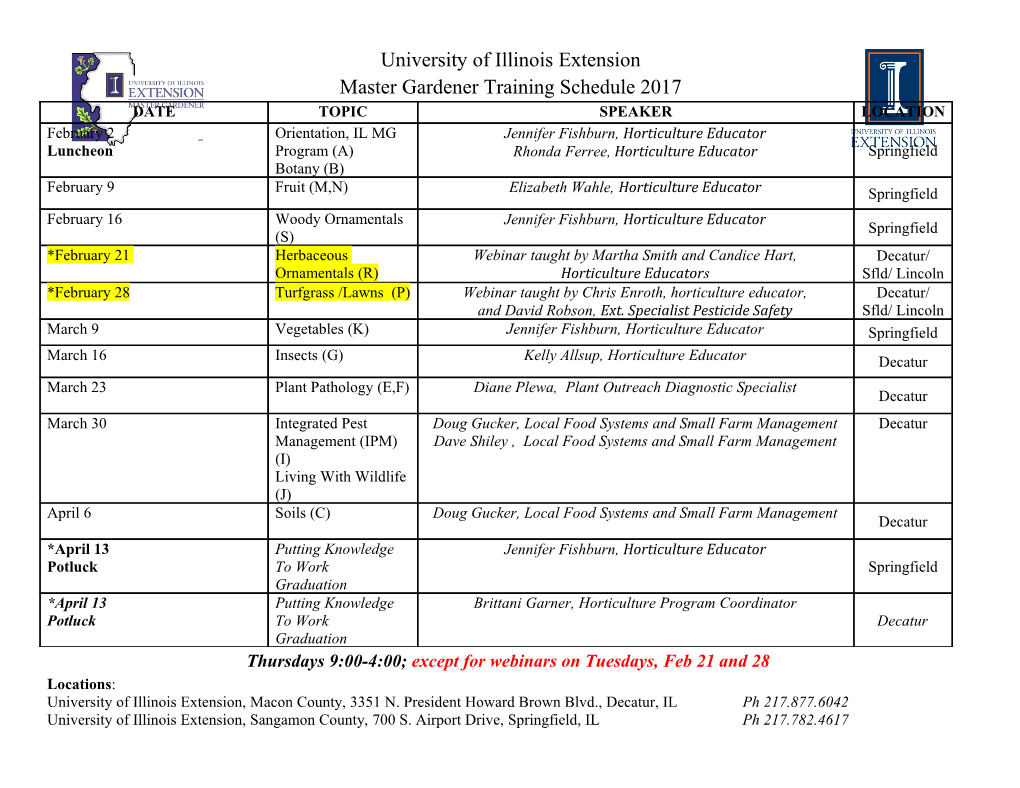
Physics and Performance of Ultra-High-Frequency Field-Effect Transistors Frank Schwierz Technische Universität Ilmenau, Germany Outline • Introduction • RF FET Physics and Design Rules • Performance of RF FETs • Options for Future RF FETs • Conclusion IEEE Electron Device Colloquium Orlando, Feb 21-22 2008 1 What Does Ultra-High Frequency Mean? In this talk: Ultra-High-Frequency = much above 1 GHz Synonymous: - microwave electronics - RF (radio frequency) electronics. In the follwing: ultra-high-frequency = RF Traditionally: - defense related applications clearly dominated Currently: - large consumer markets for RF products - defense related applications Spectrum of civil RF applica- tions, Ref. ITRS 2005. Currently most civil RF applications with large- volume markets operate below 10 GHz. Future applications at higher frequencies are envisaged. 2 Are There Applications Beyond 94 GHz? The THz gap: 300 GHz … 3 THz. Output power of RF sources Ref.: D. L. Woolard et al., Proc IEEE Oct. 2005. Examples for applications in the THz gap: - ultrafast information and communication technology - security (detection of weapons and explosives) - medicine (e.g. cancer diagnosis) -… RF transistors providing useful gain and output power in the THz gap are highly desirable. 3 RF Electronics vs. Mainstream Electronics Mainstream electronics (processors, ASICs, memories) Semiconductors Transistor Types • Si • MOSFETs • For a few applications BJTs RF electronics Semiconductors Transistor Types • III-V compounds based • MESFET - Metal Semiconductor FET on GaAs and InP • HEMT - High Electron Mobility Transistor • Si and SiGe • MOSFET - Metal Oxide Semiconductor FET • Wide bandgap materials • HBT - Heterojunction Bipolar Transistor (SiC and III-nitrides) • BJT - Bipolar Junction Transistor 4 RF FET Types For ultra-high-frequency relevant: HEMT and MOSFET Common feature - layer sequence in the active region: Gate 2DEG Channel – gate - barrier Barrier Source Drain - 2DEG channel - substrate Substrate HEMT Barrier 2DEG Channel Gate (SiO 2) Source Drain Substrate Si MOSFET 5 RF FET Types 2DEG EC EC ∆ E Band diagram of an AlGaAs/InGaAs EF EC F EC heterojunction as used in GaAs EV EF pHEMTs ∆ EV EV EV AlGaAs InGaAs AlGaAs/InGaAs Gate Source Drain n+ cap n+ cap Schematic of a HEMT Barrier L High Electron Mobility Transistor Barrier / buffer Substrate 2DEG channel Channel layer 6 RF FET Types FET Type Barrier Channel Substrate Conventional GaAs HEMT AlGaAs GaAs GaAs GaAs pHEMT AlGaAs In xGa 1-xAs GaAs (p = pseudomorphic) x ∼∼∼ 0.2 GaAs mHEMT InAlAs In xGa 1-xAs GaAs (m = metamorphic) x ≥≥≥ 0.53 InP HEMT InAlAs In xGa 1-xAs InP x ≥≥≥ 0.53 GaN HEMT AlGaN GaN SiC, Sapphire, Si Si MOSFET SiO 2 Si Si 7 Physics and Performance of Ultra-High-Frequency Field-Effect Transistors Outline • Introduction • RF FET Physics and Design Rules • Performance of RF FETs • Options for Future RF FETs • Conclusion IEEE Electron Device Colloquium Orlando, Feb 21-22 2008 8 RF Transistor Figures of Merit P Power gain G G = out General definition: Pin In RF electronics - several power gain definitions. Frequently used: unilateral power gain U. y − y 2 U = 21 12 []()()()()− 4 Re y11 Re y22 Re y12 Re y21 Current gain h i y 21 h = 2 = 21 General definition: 21 i1 y11 Note: gains are [ ] = ( ) usually given in dB Power Gain dB 10 log Power Gain [ ] = h21 dB 20 log h21 9 The Characteristic Frequencies f T and fmax 50 h21 and U of a GaAs RF FET U (-20 dB/dec) After K. Onodera et al., TED 38, p. 429. 40 h21 and U roll off at higher f =138 GHz frequencies at a slope of approx. 30 max h (-20 dB/dec) 21 –20 dB/dec. Cutoff Frequency f Gain (dB) Gain 20 T f =108 GHz Frequency, at which the magnitude Measured h T 21 of h21 rolls off to 1 (0 dB). 10 Measured U Max. Frequency of Oscillation fmax 0 Frequency, at which U rolls off 0.1 1 10 100 to 1 (0 dB). Frequency (GHz) Rule of thumb: ××× - fT should be 5 … 10 the operating frequency f op of the system in which the transistor is to be used. - fmax should be around or larger than f T. All discussions in this talk will be restricted to f and f . T max 10 Evolution of f T and f max 3000 * InP HBT InP HEMT InP HEMT Evolution of 1000 GaAs pHEMT fT and f max AlGaAs/GaAs HEMT • fT and f max have GaAs MESFET InP HBT been increased 100 GaAs mHEMT continuously. InP HEMT (GHz) Ge BJT • Record performance T f AlGaAs/GaAs HEMT of RF FETs (Feb'08): , max f 10 - fT 610 GHz f max Si BJT Yeon et al. IEDM 2007 fT *Transferred substrate - fmax 1.2 THz 1 Lai et al. IEDM 2007 1960 1970 1980 1990 2000 2010 Year Research priority for RF transistors defined in the 2007 ENIAC SRA: Extend III/V and SiGe technologies up to 1 THz. 11 RF FET Physics and Design Rules Traditional opinion: • Si is a "slow" material, Si MOSFETs are slow. • III-Vs are "faster" materials and III-V HEMTs are fast. • Key to get a fast FET: - make the gate as short as possible, - take the material with the highest mobility for the channel. We will clarify if this traditional opinion is still correct. 12 RF FET Physics and Design Rules – Intuitive Approach - How to make a FET fast? RF FETs have to react fast on variations of the input signal Gate more positive VGS VG (gate-source voltage V GS ). The charge distribution in the Source Drain the channel has to be changed n+ L n+ fast. To achieve this we have to less positive VGS consider Transistor design - Short channel, small L n+ n+ - Fast carriers (n-channel) - Minimized parasitics. Material issues These rules are helpful but we need - Fast carriers (high to take a closer look on FET physics ! mobility, high velocity). 13 RF FET Physics and Design Rules – More Detailed Approach - R Cgd R Methodology G D Gate Drain Cgs rds Equivalent FET Circuit gmvGS Ri Expressions for f T and f max RS How do the circuit elements Source affect f T and f max Influence of - design features, dimensions Compare different - material properties RF FET types on circuit elements, f , f T max 14 FET Equivalent Circuit C RG gd RD Gate Drain Approximations Cgs rds for f T and f max gmvGS R gm i )1( f ≈ T π 2 Cgs R S v )2( f ≈ T 2 π L Source g 1 )3( f ≈ m T π []+ []+ ()+ + ()+ 2 Cgs Cgd 1 gds RS RD Cgd gm RS RD f )4( f ≈ T max []()+ + + π 2/1 2 gds Ri RS RG 2 fT RG Cgd (1), (3), and (4) suggest: - gm should be large - all other elements should be small 15 Transconductance and Gate-Source Capacitance (1) C RG gd RD I = q n v W Gate Drain D sh Cgs rds d I d n g v = D = sh m GS gm q v W R i d VGS d VGS d Qch d nsh RS = − = Cgs q L W d VGS d VGS Source Large g m: - large dn sh /dV GS , large v. Small C GS : - small L. - BUT: for a given gate length we need a LARGE intrinsic C GS . 16 Carrier Transport Slope near the origin µ o1 Stationary velocity- Maximum velocity field characteristics vmax1 y t i 3 Figures of Merit c o l e - low-field mobility µ0 v n Material 1: III-V - maximum velocity v max o µ r o2 t - critical field E c v c e max2 l E Material 2: Si Desirable for high g m - high µ0 - high v max E E Electric field c1 c2 - small E c 17 Carrier Transport (Low-Field) 10 5 InSb Low-field mobility vs bandgap (undoped bulk material, 300 K) / Vs) 2 InAs Trend: High mobility – narrow bandgap In Ga As 0.53 0.47 10 4 GaAs Problems of narrow In Ga As 0.2 0.8 InP bandgap materials In Al As - low breakdown field 0.52 0.48 - high intrinsic carrier Al Ga As 0.3 0.7 concentration Electron low-field mobility (cm GaN Si ∝ − EG 10 3 ni exp 0 1 2 3 4 2 kB T Bandgap (eV) Intermediate conclusion regarding µo: narrow/medium bandgap III-Vs have an edge. 18 Carrier Transport (High-Field) 5 InAs 3.0 In Ga As 2.5 GaN .53 .47 4 2.0 GaAs Trend: cm/s) 7 1.5 GaN cm) v(10 1.0 7 Narrow bandgap – high v Si max 3 0.5 and small E 0.0 c 0 100 200 300 400 Field (kV/cm) 2 Intermediate conclusion Drift velocity (10 1 regarding v max and E c: III-Vs have an edge. 0 0 20 40 60 80 100 Electric field (kV/cm) Velocity-field characteristics 19 Gate Modulation Efficiency (dn sh /dV GS ) • Gate modulation efficiency is related to the density of states DOS in the conduction band • The DOS is related to the effective mass Material m* DOS (bulk) Si 1.18 InAs 0.031 In 0.53 Ga 0.47 As 0.048 GaAs 0.067 • Large m* DOS – large gate modulation efficiency Intermediate conclusion regarding DOS: Si has an edge. 20 Transconductance and Gate-Source-Capacitance (2) g g )1( f ≈ m = m T π π ()+ 2 Cgs 2 Cgs ,int Cgs , par ε = d nSh ≈ bar L W Cgs ,int q L W d VGS dbar Cgs,par : fringing, stray, pad capacitance components g ≈ m Desirable: C gs,int >> C gs,par fT ε L W i.e., large εεε /d π bar + bar bar 2 Cgs , par dbar Transistor Type Barrier εεε /d (1/nm) r,bar bar Intermediate conclusion GaAs pHEMT AlGaAs 12/30 ≈≈≈ 0.4 ≈≈≈ regarding C -C : InP HEMT In 0.52 Al 0.48 As 12.7/15 0,85 gs,int gs,par ≈≈≈ Si MOSFET SiO 2 3.9/1 4 Si MOSFETs have an edge.
Details
-
File Typepdf
-
Upload Time-
-
Content LanguagesEnglish
-
Upload UserAnonymous/Not logged-in
-
File Pages50 Page
-
File Size-