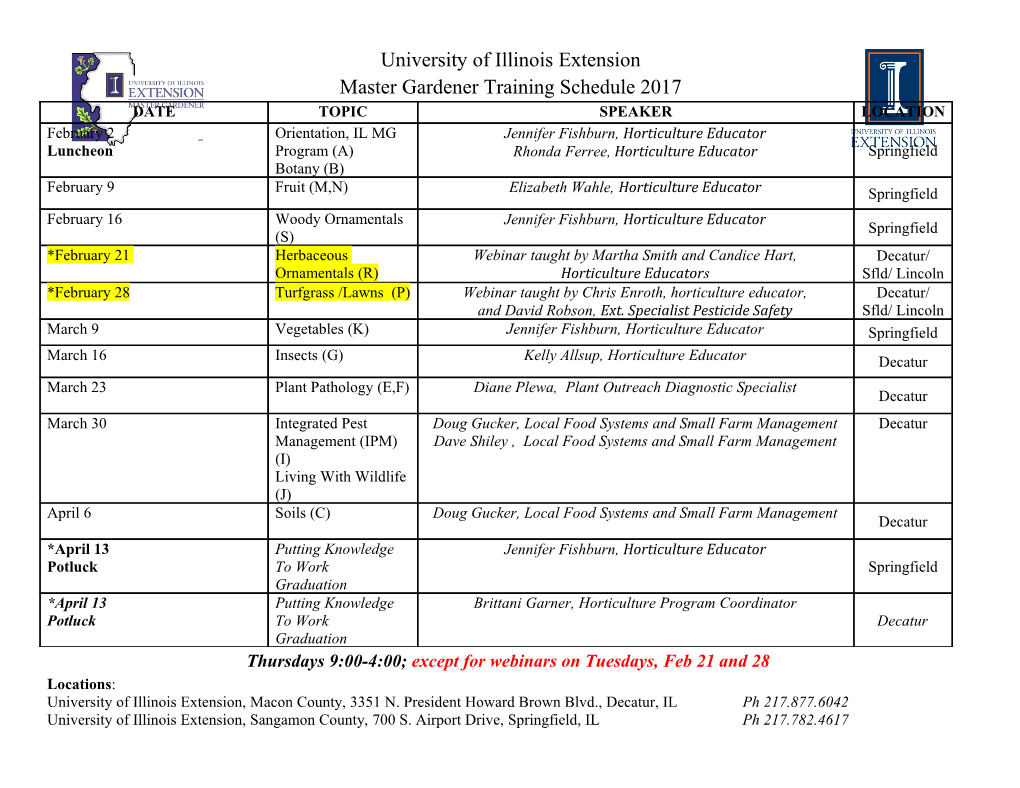
Imaging with Neutrons: The Other Penetrating Radiation Glen MacGillivray* Nray Services Inc., 729 Black Bay Road, Petawawa ON K8H 2W8 CANADA ABSTRACT Neutron radiography is a well-known imaging technique among those working at nuclear research facilities. However, the move from laboratory to industry has been hampered by the generally large neutron flux requirements and by the relatively small number of nuclear research reactors. The development of imaging techniques that require lower total neutron exposure than traditional methods, coupled with improvements to non-reactor neutron sources suggests that broader application of neutron radiology may be imminent. Keywords: neutron, imaging, radioscopy, radiography, attenuation, contrast, sensitivity, resolution, gadolinium, scintillation 1. INTRODUCTION Neutron radiography has existed as a testing technique and as a research tool for over 60 years and has grown in use and application throughout that time. Furthermore, the general location of nuclear research reactors at universities and national laboratories has ensured the availability of researchers with widely varied backgrounds and interests. An active international community sees approximately 50-100 papers published each year. The majority of publications are in refereed conference proceedings and journals. There are two on-going series' of international technical meetings (The World Conferences on Neutron Radiography and the International Topical Meetings on Neutron Radiography). Standards are being actively developed at the national and international levels that support the commercial application of neutron radiography. A society has been formed among neutron radiographers worldwide. The technology is ready for widespread application with a substantial worldwide capability for the production of neutron radiographs and with a reasonably large and organized body of professionals to support it. Nevertheless, commercial application of neutron imaging has generally lagged the technical developments in the field, with the result that there are remarkable developments that are not being exploited commercially--yet. This lag results from the generally small number of locations at which this non-destructive testing technique and research tool can be practiced. There are few sites in the world where organizations are using their own neutron radiographic equipment to inspect parts of their own manufacture. Application at the industrial scale has been largely limited to the examinations of small, high-value parts that can be shipped to one of the reactor sites where commercial neutron radiography is conducted or to one of several military sites. Broader application than this requires either a proliferation of service organizations or of in-house inspection systems, or of both. Either of these requires a cost-effective neutron source. Requirements upon neutron sources are being lessened as imaging technology improves. When high-energy neutrons are used, neutron-source costs and complexity drop dramatically, although the scope of application changes substantially as well. The availability of high-yield neutron sources based upon proton/deuteron accelerators and metal (Li, or Be) targets may encourage the proliferation of facilities that will move neutron imaging ahead of other non-destructive testing techniques and into the commercial mainstream. Imaging with neutrons involves one of: radiography, where images are produced on film; radioscopy, where digital images are prepared; and tomography, where third-dimensional data are calculated and presented; and radiology, which we will consider to be the general term. The developments foreseen in neutron radiology derive from research being conducted in areas such as: Light-emitting scintillation screens for thermal neutrons, Proton recoil scintillators for fast-neutron imaging RFQ (radio-frequency quadrupole proton accelerators, Neutron beam guides, and Accelerator targets (lithium and/or beryllium). *Correspondence: Web: www.nray.com; Telephone: (613)687-7009; Fax: (613)687-7039 Additionally, this technique is benefiting from the explosion of more generally known technologies, including: High -quantum-efficiency CCD (charge-coupled device) cameras, Laser-stimulated-readout imaging plates, Inexpensive computing power, for: Computed tomography, Monte Carlo design simulation, Image manipulation (contrast-stretching, background subtraction, etc.), and Shielding calculations. Writable and Re-writable compact discs, Large hard-disc devices and inexpensive memory, and High-speed data transmission. Neutron imaging, with a small number of noteworthy exceptions, has largely remained a solution in search of problems. It has been generally perceived as expensive and inaccessible. This paper introduces the reader to the available neutron sources, to the contrast available from neutrons of various energies (including a comparison with photons) and to the imaging techniques that are being employed. Discussed in the paper are the implications upon application development and feasibility of the existing technology. Areas of potential application growth are explored 2. NEUTRON SOURCES Neutrons have generally been most readily available in quantity at nuclear research reactors. However, the number of research reactors has been steadily declining worldwide for some years. Other sources of neutrons do exist, and may have useful special applications. This section describes, in very general terms, the neutron sources that are available today, and indicates where these sources might find application. 2.1 Nuclear Reactors Nuclear reactors represent an abundant source of thermal neutrons. Neutron flux at the entrance of a neutron collimator can be on the order of 1014 n/cm2⋅s. After collimation with a pinhole or divergent collimator, typical neutron flux at the image plane is generally in the 106 - 107 n/cm2⋅s range. This provides a good balance of depth of field (through collimation ratios on the order of 100) and speed, with exposure times to high-resolution X-ray film of several minutes to several tens of minutes. Nuclear reactors can also be fitted with cold sources, filters and guide tubes to provide neutrons in the sub-thermal or cold energy range (~1 meV). A cold neutron beam from a well-designed guide tube provides a substantial benefit to the neutron imaging specialist by almost entirely eliminating unwanted radiations from the beam. There have been several first-class research reactors built in the past few years (JRR-3 in Japan and Hanaro in Korea, for example), with excellent neutron beam facilities. The scientific community in Canada, together with Atomic Energy of Canada, Limited are planning a new state-of-the-art research reactor with truly spectacular neutron-beam capabilities including: a large cold source, a well-conceived guide hall, and both cold- and thermal-neutron guides. These developments bode well for the continuing development of advanced neutron imaging techniques. A few reactors have been used for neutron imaging that have particularly high fast neutron flux1 relative to the thermal neutron flux. Initial work on fast neutron imaging was conducted at one such reactor. Most research reactors can generate epi-thermal neutron beams (1 eV - 10 keV) if suitable filters are installed. These epi-thermal beams can be tuned in energy to some extent to optimize the sensitivity to elements with resonance absorptions at particular energies. This applies equally to analytical techniques as to imaging Nuclear research reactors are not commonplace items. The high cost of building a reactor plus the enormous perceived administrative load to manage licensing , coupled with the magnitude of the assumed liability and the decommissioning and waste disposal costs make them unattractive, generally, in the private sector. 2.2 D-T tubes These neutron sources2 are based upon deuteron beams impinging upon tritiated targets with relatively low accelerating potential and fairly high current, with the resultant yield of 14 MeV fusion neutrons at a rate of up to ~ 1011 n/s. These 14 MeV neutrons are very penetrating in and of themselves and can be used directly for fast-neutron imaging. However, the high-energy neutrons that are produced can, in principle, be moderated to a lower temperature to allow epi-thermal, thermal or perhaps even cold neutron imaging. In practice though, neutrons of such high energy require large moderators and yield relatively low thermal neutron fluxes. The relative portability of systems based upon D-T tubes of this sort makes in-field application quite possible, particularly where the contrast required can be obtained with unmoderated or under-moderated (i.e., fast or epi-thermal) neutrons. In these cases, the small size of the moderator assembly yields a small, bright source of neutrons, permitting high intensity beams at the image plane at acceptably large collimation ratios. D-T tubes are being used largely for thermal neutron radioscopy, since exposure times to film would be excessive. 2.3 Accelerators Several technologies have been developed for accelerating beams of protons or deuterons. Cyclotrons, both conventional and super-conducting have been developed and are commercially available that can produce >100 mA or more of protons with energies in the 12 MeV range3. Such cyclotrons can be used for generating collimated thermal neutron beams with fluxes on the order of 106 n/cm2⋅s when used with a suitably-designed beryllium or lithium target. Similarly, radio-frequency quadrupole
Details
-
File Typepdf
-
Upload Time-
-
Content LanguagesEnglish
-
Upload UserAnonymous/Not logged-in
-
File Pages10 Page
-
File Size-