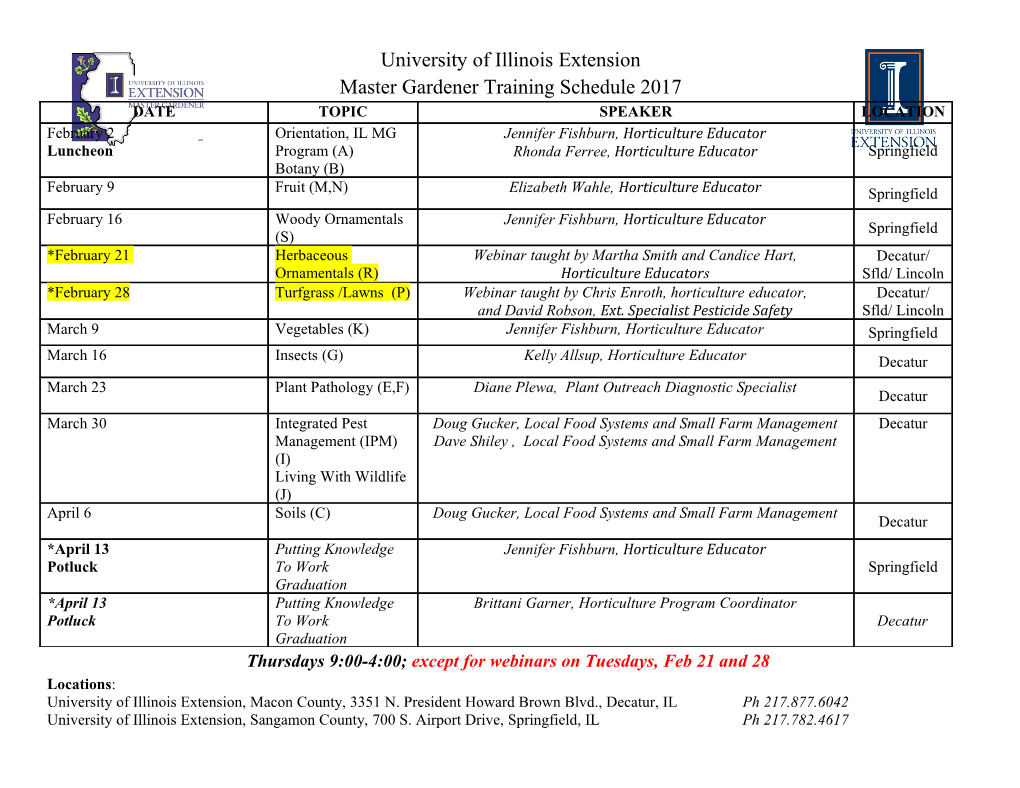
Hladky and Barrand Fluids Barriers CNS (2016) 13:19 DOI 10.1186/s12987-016-0040-3 Fluids and Barriers of the CNS REVIEW Open Access Fluid and ion transfer across the blood– brain and blood–cerebrospinal fluid barriers; a comparative account of mechanisms and roles Stephen B. Hladky* and Margery A. Barrand Abstract The two major interfaces separating brain and blood have different primary roles. The choroid plexuses secrete cerebrospinal fluid into the ventricles, accounting for most net fluid entry to the brain. Aquaporin, AQP1, allows water transfer across the apical surface of the choroid epithelium; another protein, perhaps GLUT1, is important on the basolateral surface. Fluid secretion is driven by apical Na+-pumps. K+ secretion occurs via net paracellular influx through relatively leaky tight junctions partially offset by transcellular efflux. The blood–brain barrier lining brain microvasculature, allows passage of O2, CO2, and glucose as required for brain cell metabolism. Because of high resistance tight junctions between microvascular endothelial cells transport of most polar solutes is greatly restricted. Because solute permeability is low, hydrostatic pressure differences cannot account for net fluid movement; however, water permeability is sufficient for fluid secretion with water following net solute transport. The endothelial cells have ion transporters that, if appropriately arranged, could support fluid secretion. Evidence favours a rate smaller than, but not much smaller than, that of the choroid plexuses. At the blood–brain barrier Na+ tracer influx into the brain substantially exceeds any possible net flux. The tracer flux may occur primarily by a paracellular route. The blood–brain barrier is the most important interface for maintaining interstitial fluid (ISF) +K concentration within tight limits. This is most likely because Na+-pumps vary the rate at which K+ is transported out of ISF in response to small changes in K+ concentration. There is also evidence for functional regulation of K+ transporters with chronic changes in plasma concentration. The blood–brain barrier is also important in regulating HCO3− and pH in ISF: the principles of this regulation are reviewed. Whether the rate of blood–brain barrier HCO3− transport is slow or fast is discussed critically: a slow transport rate comparable to those of other ions is favoured. In metabolic acidosis and alkalosis variations in HCO3− concentration and pH are much smaller in ISF than in plasma whereas in respiratory acidosis variations in pHISF and pHplasma are similar. The key similarities and differences of the two interfaces are summarized. Keywords: Blood–brain barrier, Choroid plexus, Brain interstitial fluid, Cerebrospinal fluid, Fluid secretion, pH regulation, Potassium regulation, Sodium transport, Potassium transport, Chloride transport, Bicarbonate transport, Tight junctions, Water channels, Paracellular transport, Transcellular transport, Ion transporters, Ion channels, Electroneutrality, Endothelial, Epithelial, Neurovascular unit, Astrocyte endfeet Table of Contents 1.2 Previous reviews 1 Background 1.3 Notation and conventions for expressing concen‑ 1.1 Overview of locations and functions of the cho‑ trations, partial pressures and other values roid plexuses and the blood–brain barrier 2 Transfers of water, O2, CO2 and major nutrients between blood and brain parenchyma *Correspondence: [email protected] Department of Pharmacology, University of Cambridge, Tennis Court Road, Cambridge CB2 1PD, UK © The Author(s) 2016. This article is distributed under the terms of the Creative Commons Attribution 4.0 International License (http://creativecommons.org/licenses/by/4.0/), which permits unrestricted use, distribution, and reproduction in any medium, provided you give appropriate credit to the original author(s) and the source, provide a link to the Creative Commons license, and indicate if changes were made. The Creative Commons Public Domain Dedication waiver (http://creativecommons.org/ publicdomain/zero/1.0/) applies to the data made available in this article, unless otherwise stated. Hladky and Barrand Fluids Barriers CNS (2016) 13:19 Page 2 of 69 2.1 Water movement at the blood–brain barrier 4.3.1 Results mainly concerned with K+ move‑ and choroid plexuses ment + 2.2 O2 and CO2 transfer at the blood–brain barrier 4.3.2 Results mainly concerned with Na move‑ and production of metabolic water ment 2.3 Importance of neurovascular coupling for O2 4.3.3 Results from further in vivo studies using and CO2 transfer at the blood–brain barrier inhibitors 2.4 Glucose and amino acid transfer at the blood– 4.3.4 Transcellular versus paracellular routes brain barrier for Na+ and Cl− 2.4.1 Glucose 4.3.5 Comparison of Na+ tracer flux, net Na+ flux 2.4.2 Amino acids inferred from tracer data and the net Na+ flux 2.5 Transfers of glucose and amino acids across the needed to support any significant fluid choroid plexuses secretion 3 Fluid secretion by the choroid plexuses 4.3.6 Results concerned with water transfer 3.1 Composition of fluid secreted by the choroid plex‑ 4.4 Evidence for the presence of ion transporters able uses to move osmotically important solutes across the 3.2 Rate of fluid secretion across the choroid plexuses blood–brain barrier 3.3 Mechanisms of fluid secretion by the choroid 4.4.1 Expression and localization of Na+, plexuses K+‑ATPase 3.4 Maintenance of nearly isosmotic fluid secretion 4.4.2 Evidence for expression of other ion by the choroid plexuses transporters at the blood–brain barrier 3.4.1 Comparisons with kidney proximal tubules 3.4.2 Transcellular and paracellular routes for water 4.5 Functional evidence of ion transport at the blood– transfer brain barrier from in vitro studies with brain 3.5 Expression of ion transporters endothelial cells 3.6 Summary of mechanisms for the principal species 4.5.1 Evidence concerning Na+, K+ and Cl− trans‑ transported port + − − + 3.6.1 Pathways of Na transport 4.5.2 Evidence concerning HCO3 , Cl and H 3.6.1.1 Is an amiloride‑sensitive ion channel involved transport in Na+ transport at the choroid plexus? 4.5.3 Evidence concerning K+ transport − − 3.6.2 Pathways of HCO3 and Cl transport 4.6 Mechanisms for ion and water movements − 3.6.3 Role of carbonic anhydrase in HCO3 trans‑ across the endothelial layer of the blood–brain port barrier: a current description 3.6.4 Pathways for K+ transport 4.6.1 Na+ transport − − 3.7 Electrical current and potential difference 4.6.2 HCO3 and Cl transport across the choroid plexus 4.6.3 Role of carbonic anhydrase in HCO3− trans‑ 4 Ion and fluid transport at the blood–brain barrier port 4.1 Evidence for and against fluid secretion by the 4.6.4 K+ transport blood–brain barrier 4.7 A description of Na+, Cl− and water transport 4.1.1 Net fluxes of inorganic ions across the across the blood–brain barrier blood–brain barrier 5 Role of the brain interfaces in regulation of K+ in the 4.2 Hydrostatic pressure gradients cannot be respon‑ brain sible for significant fluid movement across the 5.1 K+ transport across the choroid plexuses blood–brain barrier 5.2 K+ transport across the blood–brain barrier 4.3 Functional evidence for ion transport at the blood– 5.3 The role of K+ channels in glial endfeet brain barrier from in vivo (and ex vivo) studies Hladky and Barrand Fluids Barriers CNS (2016) 13:19 Page 3 of 69 − 6 pH and concentration of HCO3 in the extracellular all the hallmarks of a “leaky” secretory epithelium designed − to produce a large volume of nearly isosmotic fluid. fluids of the brain: importance of HCO3 transport at the blood–brain barrier and choroid plexuses The blood–brain barrier is in some ways a more com‑ plicated structure than the choroid plexus. It separates 6.1 Consideration of the physiological principles blood from interstitial fluid (ISF) and cells of the brain important for understanding pH regulation parenchyma. The barrier, so‑called because it greatly 6.1.1 The interrelationship of CO2, HCO3− restricts movements of many substances between brain and H+ and blood, consists of the endothelial lining of almost the 6.1.2 Constraints imposed by the principle entirety of the brain microvascular network (see Fig. 1b). However, the transfers the barrier permits are at least as of electroneutrality and constancy of osmo‑ important as those it hinders. It is ideally placed both lality to deliver substrates for brain cell metabolism and to 6.2 Contribution of physiological buffering by brain remove the corresponding wastes. It is also important in cells to regulation of ISF pH regulating ISF ionic composition. 6.3 Impact of lactic acid production and removal The lining of the brain microvessels differs from that of on ISF pH peripheral vessels in that the endothelial cells are joined together by tight junctions that greatly restrict free, paracel‑ 6.4 Involvement of HCO3− transport across the blood– lular movement of substances (see Fig. 1d), the exceptions brain interfaces in ISF and CSF pH regulation to this being the parts of the vasculature supplying the cho‑ 6.4.1 HCO3− transport across the choroid plex‑ roid plexuses and the circumventricular organs. The perme‑ uses ability of the blood–brain barrier to ions such as Na+ and − 6.4.2 HCO3− transport across the blood–brain Cl is low, not much larger than the permeability of many barrier cell membranes (see Sect. 4). While the low passive perme‑ ability to these ions as judged from unidirectional tracer 7 Summary fluxes may reflect primarily paracellular movements, the 8 Conclusions net fluxes can still reflect transcellular transport through the 1 Background cells (see Sect. 4.3.4). The endothelial layer is surrounded by The inorganic ions in brain fluids ultimately derive from a basement membrane and pericytes all closely enveloped the peripheral circulation.
Details
-
File Typepdf
-
Upload Time-
-
Content LanguagesEnglish
-
Upload UserAnonymous/Not logged-in
-
File Pages69 Page
-
File Size-