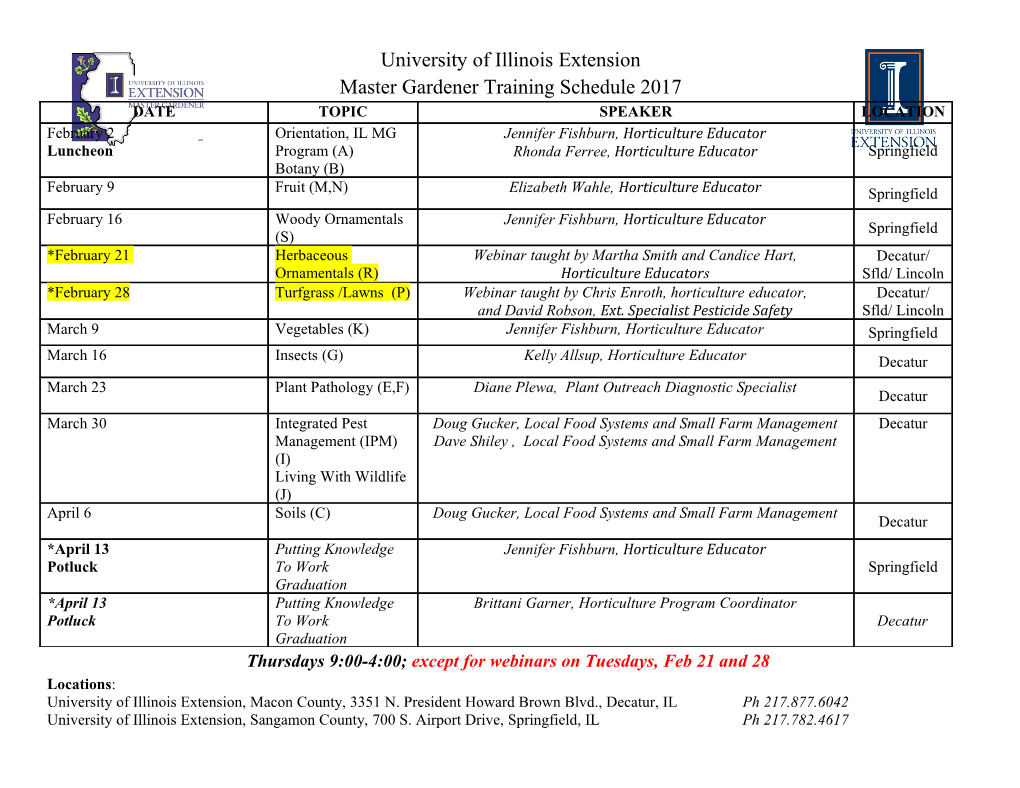
The Chemical Consequences of Cavitation Kenneth S. Suslick School of Chemical Sciences, University of Illinois at Urbana-Champaign, 600 S. Mathews Av., Urbana, IL 61801 USA Acoustic cavitation is responsible for both sonochemistry and sonoluminescence. Bubble collapse in liquids results in an enormous concentration of energy from the conversion of the kinetic energy of liquid motion into heating of the contents of the bubble. Sonoluminescence has provided a detailed spectroscopic probe of the conditions created during cavitation. the collapse of bubbles in a multi-bubble cavitation field produces hot spots with effective temperatures of ~5000 K, pressures of ~1000 atmospheres, and heating and cooling rates above 1010 K/s. In single bubble cavitation, conditions may be even more extreme.The high local temperatures and pressures, combined with extraordinarily rapid cooling, provide a unique means for driving chemical reactions under extreme conditions. A diverse set of applications of ultrasound to enhance chemical reactivity has been explored, with important applications in mixed phase synthesis, materials chemistry, and biomedical uses. Ultrasound does not drive chemical reactions mean that ultrasound provides a unique mechanism for through a direct coupling of the acoustic field with generating high energy chemistry. Figure 1 presents an chemical species on a molecular level. Instead, interesting comparison of the parameters that control sonochemistry and sonoluminescence are primarily the chemical reactivity (time, pressure, and energy) for chemical consequences of acoustic cavitation: the various forms of chemistry. formation, growth, and implosive collapse of bubbles in liquids irradiated with high-intensity ultrasound. Bubble collapse during cavitation serves as an effective means of concentrating the diffuse energy of sound. Compression of a gas generates heat. When the compression of bubbles occurs during cavitation, heating is more rapid than thermal transport, creating a short-lived, localized hot spot. Emission of light often accompanies sonochemistry. Such sonoluminescence provides an extremely useful spectroscopic probe of the conditions created during cavitation bubble collapse. As with sonochemistry, sonoluminescence is a consequence of acoustic cavitation. Thus, cavitation can create extraordinary physical and chemical conditions in otherwise cold liquids. SONOCHEMISTRY FIGURE 1. Chemistry: the interaction of energy and matter. Chemistry is fundamentally the interaction of energy and matter. Chemical reactions require energy in one form or another to proceed: chemistry stops as There is a wide range of chemical and physical the temperature approaches absolute zero. Chemists consequences that high intensity can induce, as shown have only limited control, however, over the nature of schematically in Figure 2. The chemical effects of this interaction. In large part, the properties of a ultrasound can be divided into three general types: specific energy source determine the course of a ‘homogeneous’ liquids, heterogeneous liquid-liquid, and chemical reaction. Ultrasonic irradiation differs from heterogeneous liquid-solid systems [1]. Sonocatalysis, traditional energy sources (such as heat, light, or i.e., the use of ultrasound to initiate or modify catalytic ionizing radiation) in duration, pressure, and energy reactions, overlaps these classes. It is worth noting that per molecule. The immense local temperatures and in the great diversity of sonochemical phenomena, pressures together with the extraordinary heating and sonoluminescence is only one small (but cooling rates generated by cavitation bubble collapse mechanistically useful) piece in a larger scheme [2]. Primary Acoustic Phase: Gas Supercritical Fluid are of special interest because their properties are often Gas/Gas Gas/Liquid Gas/Solid SCF SCF/Solid Primary/Secondary Phases: distinctly different than either molecular species or bulk Atomization Heterogen. Extraction Catalysis materials. Application Classes: Catalysis Polymer Defoaming Surface Hydrogenation Modification Hydrocarbon Partial Oxidation Solids Communition Dispersion/ M(CO)M(CO)x(NO)(NO)y Delivery x y Primary Acoustic Phase: Liquid Solid Welding, Grinding, Cutting SupportedSupported ULTRA- SOUND NanophaseNanophase Primary/Secondary Phases: Liquid/Gas Liquid/Liquid Liquid/Solid Fractoluminescence (cavitation) Fatigue Fracture MetalMetal inorganic MetalMetal CatalystsCatalysts support ColloidsColloids stabilizer "Homogeneous” Extraction With Without Application Classes: Sonochemistry Cavitation Cavitation Mixed Phase Water Nanophase Reactions Mixed Phase Sono- Enhancement of M n Cleaning Mass Transport Remediation Inorg Materials Reactions Catalysis Sulfur Sono- Emulsification luminescence Degreasing Dissolving Hydrogenation source Metal Reactions oxygen Microelectronics Hydrocarbon Rural Catalysts, Wire cleaning Oxidation- Partial Oxidation NanophaseNanophase NanophaseNanophase ground Magnetic Microencap- Reduction Rxns. water Materials sulation MetalMetal MetalsMetals Oxides hydro- Sulfides Oxides carbon Sulfides FIGURE 2. Classification of the chemical and physical NanophaseNanophase Metals, Alloys, effects of ultrasound. Metals, Alloys, n = 100 - 1000 & Carbides & Carbides The divisions of sonochemistry reflect both the type of chemical reactions observed and the physical FIGURE 3. Sonochemical synthesis of nanostructured mechanisms underlying them. Homogeneous materials. sonochemistry (a misnomer, since cavitation is by definition two phases) of liquids reflects the cavitation Another important application of sonochemistry to hot spot most directly in its high energy chemistry. materials chemistry has been in the preparation of Liquid-liquid reactions are generally due to increase biomaterials, most notably protein microspheres [6, 7]. mass transport between the two liquid phases, as Using high intensity ultrasound and simple protein expected for emulsions. Although length limitations solutions, a remarkably easy method to make both air- prevent a detailed discussion here, the role of ultrasound filled microbubbles and nonaqueous liquid-filled in increasing reactivity in liquid-solid systems has microcapsules has been developed (Figure 4). The proved extremely important in chemical synthesis mechanism responsible for microsphere formation is a [1,3,4]. combination of two acoustic phenomena: Cavitation has dramatic effects on the reactivities emulsification and sonochemistry. The former of solutions containing either extended solid surfaces provides uniform size dispersion and the latter the HO2 or fine powders. Cavity collapse near an extended solid necessary for forming inter-protein disulfide surface becomes non-spherical, drives high-speed jets of crosslinks. The microspheres can be intravenously liquid into the surface, and creates shockwave damage injected to pass unimpeded through the circulatory to the surface. This process can produce newly exposed, system. Consequently, these protein microspheres have highly heated surfaces and is responsible for the a wide range of biomedical applications, including erosion/corrosion problems associated with their use as echo contrast agents for sonography, as hydrodynamic cavitation. Furthermore, cavitation and magnetic resonance imaging contrast agents, and for the shockwaves produced during ultrasonic irradiation drug delivery, among others [6,8]. of liquid-powder slurries can accelerate solid particles to high velocities [5]. The interparticle collisions that result are capable of inducing striking changes in surface morphology, composition, and reactivity. Thus, microjet and shock wave impact (on large surfaces) and interparticle collisions (with powders) have substantial effects on the chemical composition and physical morphology of solids that can dramatically enhance chemical reactivity. Over the past few years, the synthesis of inorganic materials has developed as one of the most important applications of sonochemistry [6]. Cavitation provides a unique set of conditions to produce unusual materials from precursors dissolved in solution. In addition, it provides facile access to nanometer sized particles of FIGURE 4. Scanning electron micrograph of various solids (Figure 3). Such “nanophase” materials sonochemically prepared protein microspheres. · · SONOLUMINESCENCE from excited states of CO2, CH , and OH is observed, again similar to flame emission. As with sonochemistry, sonoluminescence derives Most recently, we have extended our spectral from acoustic cavitation. There are two classes of analysis approach to excited metal atom MBSL sonoluminescence [2]: multiple-bubble sonolumine- spectra. Ultrasonic irradiation of volatile scence (MBSL) and single-bubble sonoluminescence organometallics (such as Fe(CO)5 or Cr(CO)6) in a low (SBSL). Cavitation is a nucleated process, and liquids volatility organic liquid produces intense sonolumine- generally contain large numbers of particulates that scence that corresponds to the known atomic emission serve as nuclei. Consequently, the “cavitation field” lines of the metals [12], again analogous to flame generated by a propagating or standing acoustic wave emission. Hot spot temperatures are sufficient not only typically consists of very large numbers of interacting to dissociate all the CO ligands from the metal bubbles, distributed over an extended region of the complex, but also to produce excited state metal liquid. If this cavitation is sufficiently intense to atoms. Figure 5 shows a
Details
-
File Typepdf
-
Upload Time-
-
Content LanguagesEnglish
-
Upload UserAnonymous/Not logged-in
-
File Pages33 Page
-
File Size-