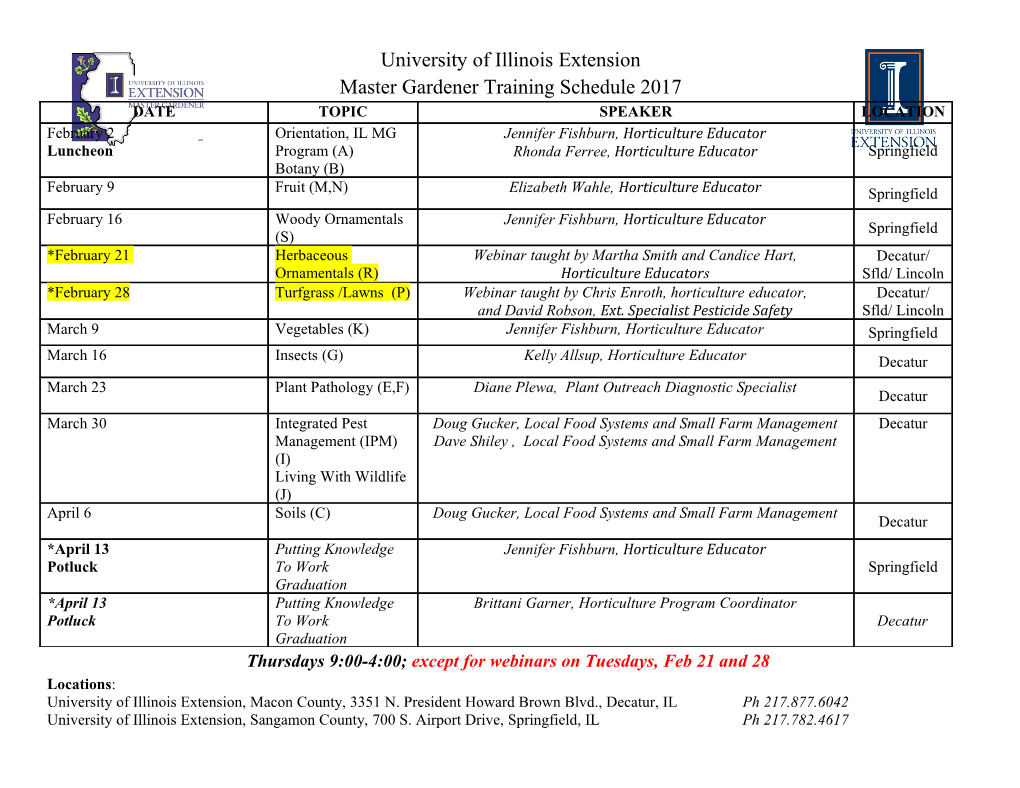
Note: this document may contain some elements that are not fully accessible to users with disabilities. If you need assistance accessing any information in this document, please contact [email protected]. EPA Hydraulic Fracturing Workshop March 10th - 11th, 2011 “Fracture Design in Horizontal Shale Wells – Data Gathering to Implementation” Tim Beard Sr. Engineering Advisor - Completions Chesapeake Energy Corporation 6100 N. Western Avenue ı Oklahoma City, OK 76118 ı 405-935-8000 [email protected] ı chk.com ı NYSE: CHK 2 Presentation Overview Goal Planning • Data • Measuring • Validating • Properties Needed in Modeling • Designing the Hydraulic Fracture • Frac Models in Vertically Heterogeneous Formations Execution 3 CHK’s Operating Areas Low-risk, U.S. onshore asset base; Not exposed to economic, geopolitical or technological risks internationally or in the Gulf of Mexico 4 Shale Information Shale Play Fayetteville Barnett Eagle Ford Haynesville Marcellus Average Depth From 4,500 7,400 9,000 11,500 7,100 Surface (ft) Bottom Hole 130 190 260 320 145 Temperature (F) Bottom Hole 2,000 2,900 6,200 10,000 4,600 Pressure (psi) 5 What is the Goal of Hydraulic Fracturing? Maximize the Stimulated Reservoir Volume (SRV) along the horizontal wellbore for a given well spacing to maximize hydrocarbon production within the zone of interest. Orientation and lateral length Vertical placement within flow unit Rock Properties/Mechanics Stages/Perf Clusters/Isolation Fluid and proppant selection 6 Planning 7 What data do we use? What are the main variables that need to be factored into each frac design? • Porosity and Permeability • Maximum Principle Stress Direction • Lateral Length • Lithology of Pay • Brittleness vs. Ductility • Young’s Modulus • Stress Anisotropy • Poisson’s Ratio • Natural Fractures • Fracture Toughness • Gas or Liquids Reservoir • Thickness • Temperature • Barriers • Reservoir Pressure • Depth • In-Situ Stress 8 Wireline Log Data Logs are run in regionally representative pilot wells over the zone(s) of interest. Triple Combo Log, Spectral Gamma Ray, Dipole Sonic Log, Formation MicroImager The data gathered from the logs is utilized to do a petrophysical analysis and to calculate the rock mechanical properties of the reservoir to determine pay intervals, barriers, etc. FMI and multi-caliper log data are also used to determine a maximum and minimum principle stress direction and to determine if there are natural fractures present. 9 Lateral Orientation Maximum Principle Stress Direction ● Perpendicular to maximum principle stress ● Optimize transverse fracturing ● Slight variations for more efficient pattern development Lateral Placement 10 Lateral PlacementComposite Well: PROCKO_MARTIN_WHITMAN_625599 COMPANY: CHK DATUM FOR ELEVATION: GR LOCATION: TWP - Range - Sec SURFACE ELEVATION: 15.00 LATITUDE: 39.0000 MEASUREMENT REF.: LONGITUDE: 80.0000 ELEVATION MEAS. REF.: - X COORDINATE: 1745180.06 DRILLED DEPTH: 7780.00 Y COORDINATE: 14416529.67 VERTICAL UNITS: FEET HORIZONTAL UNITS: FEET DATE LOGGED: 31-Jul-2007 DATE PLOTTED: 27-Oct-2008 VERTICAL SCALE: 1:240 CPHI_GAS_1 DEPTH (FEET) RM RMC 20 V/V 0RMF DFD BHT SHT BIT SIZE CPHI_1 7120.00-7429.00 - @ - - @ - 20 V/V 0 - @ - - - - - RESERVOIR_INT.VOL_TOC_AM pign kintx RESERVOIR_INT.CKPERM_AM RESERVOIR_INT.RHOBC_AM RESERVOIR_INT.VCLAY_AM 1.e-06 MD 1 0.2 V/V 0 RESERVOIR_INT.CKPERMH PEFZ_1 VP_TOC_2 RESERVOIR_INT.BVH_AV KH_PRO_1 RESERVOIR_INT.SW_AV CORE_TRA.SAMPLE_ID 10 B/E 0 0 V/V 40 1.e-06 B/E 1 RESERVOIR_INT.NET HDRA_1 TREATMENT.STAGE TENS_1 crhob VP_TOC_1 PERM_DENONLY_1 0.75 G/C3 -0.25 10000 LBF 3000 2.18 FEET 2.88 0 V/V 0.4 1.e-06 MM2/MS 1 GR_1 RLA5_1 CDEN_1 VOL_TOC_3 CKPERM_3 0 GAPI 200 PERFS.PERFS 0.2 OHMM 2000 2.18 G/C3 2.88 0 V/V 0.4 1.e-06 B/E 1 TOTAL_GAMMA_1 RLA4_1 RHOBC_4 vol_toc CSO_1 cperm ● Target highest quality rock with 0 GAPI 200 0.2 OHMM 2000 2.18 G/C3 2.88 0 V/V 0.4 0 F/MN 100 1.e-06 V/V 1 DCAL_1 RLA3_1 RHOZ_1 PHIE_3 VP_VCLAY_1 suwi CPERM_COMPOSITE_1 0 IN 10 0.2 OHMM 2000 2.18 G/C3 2.88 0.2 V/V 0 0 V/V 100 1 V/V 0 1.e-06 V/V 1 HCAL_1 DEPTH RLA2_1 NPHI_N_3 PERFS.COMPLETIONPETRO.FORMATIONBVH_RHOB_TRS_3 vcl_grlim CSW_1 CPERM_12_20_1 4 IN 24 FEET 0.2 OHMM 2000 0.3 MV -0.1 0.2 V/V 0 0 V/V 1 100 V/V 0 1.e-06 V/V 1 bs RXO8_1 NPHI_1 BVH_3 VCLAY_3 SW_3 CPERM_1 consideration given to stress profile 6 IN 16 0.2 OHMM 2000 0.3 V/V -0.1 0.2 V/V 0 0 V/V 1 1 V/V 0 1.e-06 V/V 1 7120.0 4 5 6 7 98 10 11 12 and fracture geometries 13 1514 16 7150 1817 19 20 21 22 2423 2625 27 2928 T_FLOW UNIT 30 31 32 3433 35 36 Preferred lateral placement in upper to 37 ● 3938 40 4241 7200 43 44 4645 47 48 middle portion of target zone to 49 50 51 5352 54 5655 57 58 59 optimize proppant placement 60 61 62 63 64 65 7250 6766 68 7069 71 72 7473 75 7776 209.0 78 2.55 0.060 0.056 0.429 0.127 0.1012 0.000484 8079 81 82 83 Toe high with option of traversing the 84 ● 8685 87 88 9089 91 7300 92 93 94 95 entire section 96 97 98 99 100 101 102 103 105104 107106 108 109 110 111 112 113 114 7350 116115 118117 119 120 122121 123 124 126125 127 128 129 130 131 T_ONONDAGA 133132 134 135 136 137 139138 7400 140 142141 143 144 145 146 148147 149 151150 T_HUNTERSVILLE 152 7429.0 153 154 11 Mechanical Properties and Stress Estimation from Acoustic Logs Elastic Moduli Estimated from Acoustic Logs Several Stress Equations are Appropriate Uniaxial Transverse Isotropic Equation (Lateral Strain Model): σHmin = (Eh/Ev)(νv/(1-νh))(σv-αPp) + 2 αPp + (Eh/(1-vh ))εhmin + (Ehvh/(1- 2 vh ))εhmax This equation expands the σtectonic to incorporate lateral strain. Tectonic strain creates greater stress in stiff sandstone/limestone beds and less stress in organic-rich shales. Estimated Stress Calibrated with Well Test 12 Pump-In Testing: Key Calibration Pump-In Tests Conventional Pump-In Tests in Cased Hole ― Closure Stress is Determined ― After Closure Analysis MDT Pump-In Tests in Open Hole ― Closure Stress is Determined Core Data The pump-in tests along with the core data calibrate mechanical properties data. 13 Fracture Model – Mechanical Properties Petrophysics processes the Dipole Sonic log for rock mechanical properties and that data is utilized in the frac model. 14 Fracture Model – Fluid Flow and Leakoff The fluid loss data input into the model. 15 Fracture Model Methodology The actual deviation survey for the well that is being modeled, as well as the planned perforations for the well, are entered. A pump schedule is entered into the fracture model. Numerous iterations with different pump schedules, perforation schemes, and other variable modifications are run to “optimize” the design. What is the play specific “optimum” design? Covers the height of the pay interval Creates a sufficiently conductive propped fracture length that fits our well and perf spacing, with some overlap. Minimizes well interference. Provides the best production results based on reservoir flow simulation. 16 Example Frac Model Results As depicted in the model, the fracture propogates primarily only in the lower stress portion of the rock. The lower stress portion of the reservoir “contains” the frac High stress barriers exist above and below the fracture matching lithology changes. Vertical variations in stress exist throughout the sections depicted. 17 Example Frac Model Results Fracture is contained by lower stress interval and high stress barriers above and below the lower stress interval. Variable stress throughout section, matching lithology changes Greater height growth typically leads to less fracture length. Note, this is predicted fluid distribution, not predicted propped fracture length. 18 Perforation Clusters and Stage Spacing 50 ’– 100’ Cluster Spacing 250 ’– 500’ Stage Spacing Not To Scale 19 Fluid Selection Utilize core data and lab fluid-rock sensitivity testing to determine fluid additives Maximize slickwater volumes vs. gelled fluid volumes Utilize light gels/crosslink to place higher sand concentrations where necessary in gas shales In liquids rich plays, more gels or crosslinked gels are utilized to promote greater conductivity in the propped fractures Reservoir modeling suggests higher primary fracture conductivity required to improve well performance CHK Promotes development and leads in the use of “greener”, more environmentally friendly hydraulic fracturing additives 20 Green Frac Status – Chemical Additives Additive Barnett Fayetteville Haynesville Marcellus Eagle Ford Friction Reducer GF* Test GF Test GF Test GF Test GF Test Biocide GF Test GF Test GF Test GF Test GF Test KCl Substitute Eliminated GF Substitute GF Substitute Eliminated GF Substitute Scale Inhibitor GF Test GF Test X X Surfactant Eliminated Eliminated Eliminated Eliminated X Gel X X Occasional X Cross-linker X X Breaker X X Occasional X HCl X X X X X * - Green Frac™ 21 Proppant Selection 100 mesh sand is typically used in the early portion of the job for enhanced distance and height, diversion, etching, and as a propping agent 40/70 and 40/80 mesh proppants are currently the predominant proppants used in gas shales 30/50 and 20/40 proppant used in some areas for fracture conductivity enhancement (especially important in the liquids rich plays) Resin Coated Tail-Ins - used where sand flowback is an issue or where more proppant strength and conductivity are needed Ceramic Proppants are utilized where higher conductivity and higher strength are required Increased proppant volumes and less fluid addresses conductivity and environmental issues 22 Execution 23 Hydraulically Fracturing the Shale Typical Hydraulic Fracturing Job: • 10-20 Pumps • 2-4 Sand Storage Units • Blender • Hydration Unit • Frac Tanks • Chemical Storage Truck • Data Monitoring Van • 20-30 Workers 24 Hydraulically Fracturing the Shale 6 layers of protection between the wellbore and groundwater aquifers during hydraulic fracturing operations.
Details
-
File Typepdf
-
Upload Time-
-
Content LanguagesEnglish
-
Upload UserAnonymous/Not logged-in
-
File Pages36 Page
-
File Size-