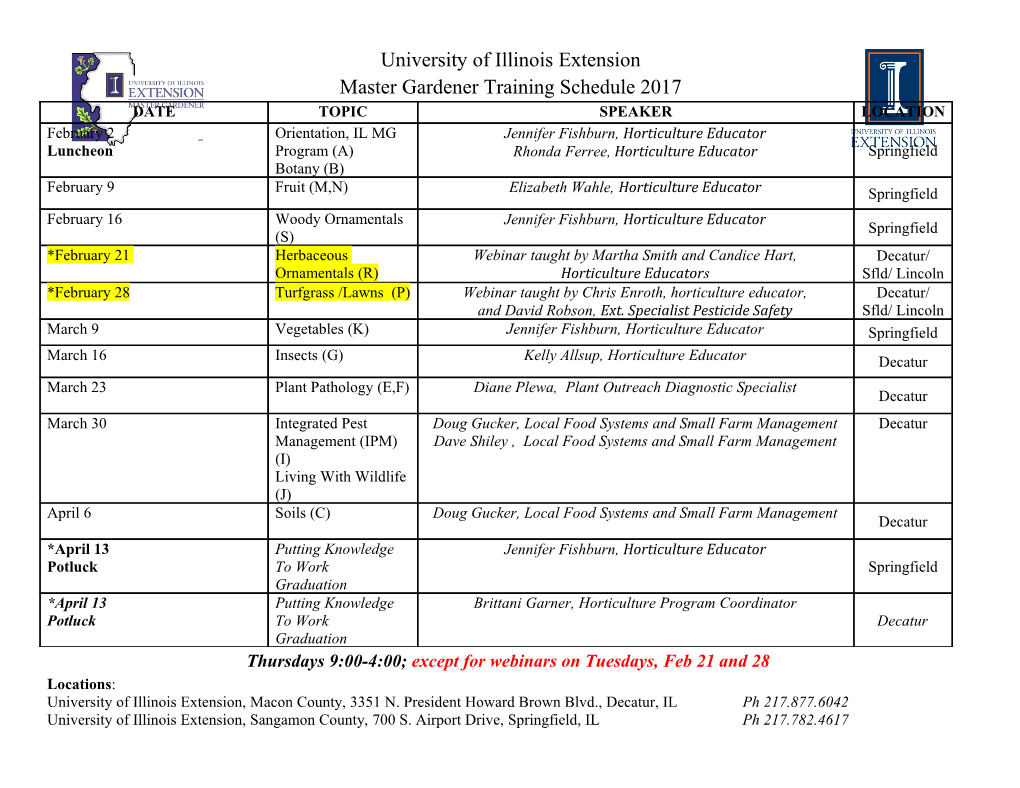
A&A 432, 585–594 (2005) Astronomy DOI: 10.1051/0004-6361:20042246 & c ESO 2005 Astrophysics Theoretical electron affinities of PAHs and electronic absorption spectra of their mono-anions G. Malloci1,2,G.Mulas1, G. Cappellini2,3, V. Fiorentini2,3, and I. Porceddu1 1 INAF - Osservatorio Astronomico di Cagliari – Astrochemistry Group, Strada n. 54, Loc. Poggio dei Pini, 09012 Capoterra (CA), Italy e-mail: [gmalloci;gmulas;iporcedd]@ca.astro.it 2 Dipartimento di Fisica, Università degli Studi di Cagliari, Complesso Universitario di Monserrato, S. P. Monserrato-Sestu Km 0,700, 09042 Monserrato (CA), Italy e-mail: [giancarlo.cappellini;vincenzo.fiorentini]@dsf.unica.it 3 INFM - Sardinian Laboratory for Computational Materials Science (SLACS) Received 25 October 2004 / Accepted 8 November 2004 Abstract. We present theoretical electron affinities, calculated as total energy differences, for a large sample of polycyclic aromatic hydrocarbons (PAHs), ranging in size from azulene (C10H8) to dicoronylene (C48H20). For 20 out of 22 molecules under study we obtained electron affinity values in the range 0.4–2.0 eV, showing them to be able to accept an additional electron in their LUMO π orbital. For the mono-anions we computed the absolute photo-absorption cross-sections up to the vacuum ultraviolet (VUV) using an implementation in real time and real space of the Time-Dependent Density Functional Theory (TD–DFT), an approach which has already been proven to yield accurate results for neutral and cationic PAHs. Comparison with available experimental data hints that this is the case for mono-anions as well. We find that PAH anions, like their parent molecules and the corresponding cations, display strong π∗ ← π electronic transitions in the UV. The present results provide a quantitative foundation to estimate the fraction of specific PAHs which can be singly negatively charged in various interstellar environments, to simulate their photophysics in detail and to evaluate their contribution to the interstellar extinction curve. Key words. astrochemistry – molecular data – molecular processes – ISM: molecules – ultraviolet: ISM – methods: numerical 1. Introduction Draine 2004). PAHs could play an important role in the forma- tion and evolution of prebiotic organic molecules (Ehrenfreund Free gas-phase polycyclic aromatic hydrocarbons (PAHs) 1999; Bernstein et al. 1999, 2003). in different charge and hydrogenation states are commonly However, despite intensive searches and some promising thought to be an important component of the interstellar candidates (Foing & Ehrenfreund 1994; Tulej et al. 1998; medium (ISM) (Duley & Williams 1981; Léger & Puget 1984; Salama et al. 1999; Ruiterkamp et al. 2002), to date no sin- Allamandola et al. 1985; Henning & Salama 1998). Such gle, specific PAH has been identified with certainty in inter- molecules have been hypothesized to efficiently absorb in the stellar spectra. Tentative identifications of neutral pyrene and visible and ultraviolet spectral ranges, producing at least a sub- anthracene were recently proposed by Vijh et al. (2004) to ac- set of the Diffuse Interstellar Bands (Léger & d’Hendecourt count for blue fluorescence observed in the Red Rectangle. 1985; van der Zwet & Allamandola 1985; Crawford et al. 1985; In our ongoing effort to produce detailed simulations of the Salama 1999) and contributing to the extinction curve (Joblin photophysics of specific PAHs (Malloci et al. 2003; Mulas et al. 1992; Joblin et al. 1992; Li & Greenberg 1997); they are fur- 2003), we are building a large database of molecular properties ffi thermore believed to e ciently convert the absorbed energy in obtained in a uniform way using available quantum-chemical infrared emission in the so-called unidentifed infrared (UIR) tools (Malloci et al. 2004), and using them as a basis to run bands (Léger et al. 1989; Allamandola et al. 1989; Salama Monte-Carlo models of their behaviour (Barker 1983; Mulas 1999; Hudgins & Allamandola 2004; Bakes et al. 2004). 1998; Joblin et al. 2002; Mulas et al. 2004). Nowadays PAHs are seen as an intermediate stage between PAHs are expected to exist in a wide variety of interstel- the gas and dust phases of the interstellar matter, i.e. large lar environments, in a complex statistical equilibrium of dif- molecules or very small grains depending on the point of view, ferent charge and hydrogenation states (Omont 1986; Bakes and, as such, they are included in some form in all interstel- et al. 2001a,b; Le Page et al. 2001, 2003). As such, any spe- lar dust models (Li & Greenberg 1997; Draine 2003; Li 2004; cific modelling of specific interstellar PAHs must take into Article published by EDP Sciences and available at http://www.aanda.org or http://dx.doi.org/10.1051/0004-6361:20042246 586 G. Malloci et al.: Electron affinities and electronic absorption spectra of PAH anions y account all the ionisation states which may be relevant for a given astronomical environment. Several studies suggested x that negatively charged PAHs play an important role in inter- z (1) (2) (3) stellar clouds (Omont 1986; Lepp & Dalgarno 1988; Salama et al. 1996; Dartois & d’Hendecourt 1997; Bakes & Tielens 1998; Bakes et al. 2001a,b), and they have thus been the sub- ject of experimental (Szczepanski et al. 1995; Halasinski et al. (4) (5) (6) (7) 2000, 2003; Ruiterkamp et al. 2002) and theoretical (Du et al. 1993; Langhoff 1996; Hudgins et al. 2000; Dessent 2000; Bauschlicher & Bakes 2000, 2001; Rienstra-Kiracofe et al. 2001, 2002; Gonzales et al. 2003; Hirata et al. 2003; Treitel et al. 2004) investigations. The most relevant molecular parameters needed to model (8) (9) (10) (11) the photophysics of interstellar PAH anions are: 1. accurate electron affinities; 2. structural parameters and vibrational analysis; 3. electronic absorption cross-sections up to the Lyman limit. (12) (13) (14) The set of anionic PAHs for which all of the above data are simultaneously available is relatively small. In particular, neither theoretical nor experimental photoabsorption spectra above ∼3 eV are available to date, to the best of our knowl- edge. The spectra of several PAH anions were taken by Shida & Iwata (1973) in organic matrices of boron, and only very few (15) (16) (17) measurements directly applicable to interstellar physical condi- tions, namely using matrix isolation spectroscopy (Szczepanski et al. 1995; Halasinski et al. 2000, 2003; Ruiterkamp et al. 2002) have been reported. Calculations of electronic excita- tion properties for PAH anions have been published for a few species and in a limited energy range (Halasinski et al. 2000, (18) (19) (20) 2003; Hirata et al. 2003). This paper aims to provide a homo- geneous, comprehensive database regarding points 1 and 3 for a large sample of PAHs, using well-known, established theo- retical techniques. We studied 22 molecules ranging in size from azu- lene (C10H8) to dicoronylene (C48H20). The PAHs considered cover an ample range of structures inside the two large classes (21) (22) of pericondensed and catacondensed species. Although non- compact PAHs are expected to be less stable than compact Fig. 1. Oriented structures of the PAHs investigated in this work: (1) azulene (C H ); (2) fluorene (C H ); (3) perinaph- ones with the same number of benzenoid rings, we extended 10 8 13 10 thenyl radical (C H ); (4) anthracene (C H ); (5) phenan- the present study to both classes, including also large linear 13 9 14 10 threne (C14H10); (6) pyrene (C16H10); (7) fluoranthene (C16H10); molecules such as pentacene (C H ). With the exception of 22 14 (8) tetracene (C18H12); (9) chrysene (C18H12); (10) C19H11 radi- naphtalene (C10H8), which is known not to exist as a bound cal; (11) perylene (C20H12); (12) benzo[g, h, i]perylene (C22H12); anion (see e.g. Rienstra-Kiracofe et al. 2001), we considered (13) anthanthrene (C22H12); (14) pentacene (C22H14); (15) coronene the same 20 PAHs we had previously studied in their neutral (C24H12); (16) dibenzo[cd,lm]perylene (C26H14); (17) bisanthrene and cationic form (Malloci et al. 2004), with the addition of (C28H14); (18) terrylene (C30H16); (19) ovalene (C32H14); (20) cir- azulene (C10H8), containing a seven-membered ring, and the cumbiphenyl (C38H16); (21) quaterrylene (C40H20); (22) dicorony- lene (C H ). C13H9 and C19H11 radicals (Hudgins et al. 2001; Weisman et al. 48 20 2001). The sample of small to medium-sized PAHs presented in this study has the same size-distribution as the mixture used by Allamandola et al. (1999) to match the mid-IR spectrum of In Sect. 2 we outline our computational approach and com- the Orion Bar. Although some studies show interstellar PAHs pare it with available experimental and theoretical data. In to be on average larger than these species (Boulanger 1999), Sect. 3 we present our results and discuss their implications in we restricted ourselves to PAHs containing less than ∼50 car- the astrophysical context, mainly showing the relation between bon atoms, since computational costs steeply increase for larger the contributions that neutral, cationic and anionic PAHs are species. The complete set of molecules investigated, together expected to give to the interstellar extinction curve. Our conclu- with their orientation, are shown in Fig. 1. sions are presented in Sect. 4, where the impact these kinds of G. Malloci et al.: Electron affinities and electronic absorption spectra of PAH anions 587 studies may have for future space-based single-molecule iden- fluorene (C13H10) and tetracene (C18H12) with the same level tifications is outlined. of theory.
Details
-
File Typepdf
-
Upload Time-
-
Content LanguagesEnglish
-
Upload UserAnonymous/Not logged-in
-
File Pages10 Page
-
File Size-