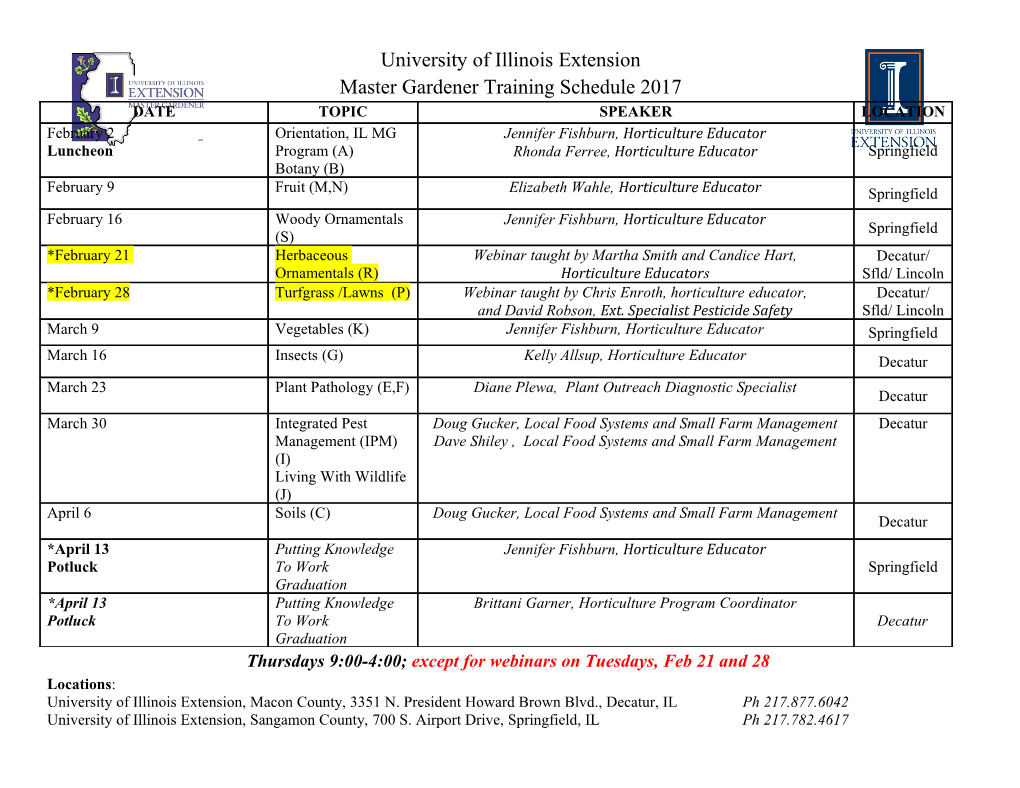
CHARACTERIZATION OF E COLI HFQ STRUCTURE AND ITS RNA BINDING PROPERTIES A thesis Presented to The Academic Faculty By Xueguang Sun In partial Fulfillment Of the Requirement for the Degree Doctor of Philosophy in the School of Biology Georgia Institute of Technology May 2006 Copyright Ó 2006 by Xueguang Sun CHARACTERIZATION OF E COLI HFQ STRUCTURE AND ITS RNA BINDING PROPERTIES Approved by : Roger M. Wartell, Chair Stephen C. Harvey School of Biology School of Biology Georgia Institute of Technology Georgia Institute of Technology Yury O. Chernoff Stephen Spiro School of Biology School of Biology Georgia Institute of Technology Georgia Institute of Technology Loren D Willimas School of Chemistry and Biochmestry Georgia Institute of Technology Date Approved: November 29 2005 To my family, for their constant love and support. iii ACKNOWLEDGEMENTS There are many people I would like to thank and acknowledge for their support and help during my five-year Ph.D. study. First and foremost, I would like to thank my advisor, Dr Roger Wartell, for his guidance and assistance throughout this chapter of my career. His constantly open door, scientific insight and perspective, and technical guidance have been integral to furthering my scientific education. He also provided knowledgeable recommendations and multi-faceted support in my personal life and bridged me to a culture which I have never experienced. Without him, it would be impossible to accomplish this thesis work. I would like to acknowledge Dr. Stephen Harvey, Dr. Yury Chernoff, Dr. Stephen Spiro and Dr. Loren Williams for being on my thesis committee and helpful discussion in structural modeling. Special thanks go out to Cameron Thomson in IPST, Dr. Andrew Lyon, Dr. Christoph Fahrni in chemistry department and Dr. Xiaodong Cheng in Emory University for readily helping me with using their fluorimeters, and Dr. Nicholas Hud and his student Swapan Jain for volunteering their time of showing me the RNA radio-labeling. On a personal note, I would like to express my appreciation to the many past and present colleagues in Atlanta and elsewhere, particularly Sixin Yan, Brooke Boudelat- Park, Bin Chen, Hyegjung Park, Hynmin Kim and other fellows in Dr Wartell’s lab for getting me through all of my Atlanta ordeals with helping hand and a friendly beverage. Finally I would like to thank my family for their eagerness to support me in any way they could. I can not begin to express the gratitude I have for my parents and siblings iv because of their incredible love and support. With visits, emails, phone calls, and joyous holiday getting together, they have inspired and encouraged me in all of my accomplishments and taught me the value of dedication, hard work, patience and faith. Throughout my life, their words of wisdom reminded me that with effort, anything is possible…even a Ph.D. v TABLE OF CONTENTS Dedication iii Acknowledgments iv List of Tables x List of Figures xi Abbreviations xiv Summary xvi Chapter I Introduction 1 Cellular distribution and synthesis of Hfq 2 Pleiotropic roles of Hfq 3 The role of Hfq in RpoS mRNA translation 4 The role of Hfq in small RNA riboregulation 5 The role of Hfq in sRNA-independent control 10 The role of Hfq in poly(A) metabolism 11 The role of Hfq in other bacteria 12 The RNA binding mechanism of Hfq 14 Objectives 17 References 19 Chapter II Structure prediction and phyletic analysis of Hfq 24 Introduction 24 Materials and Methods 26 Sequence analysis of Hfq 26 vi Secondary structure prediction of Hfq 27 Fold recognition of 3D modeling 27 Results and Discussion 28 Sequence similarity of Hfq and Sm proteins 29 Comparison of predicted secondary and 3-D structure of Hfq 30 with Sm protein Phyletic distribution of the Hfq protein 39 References 46 Chapter III Locating the surface residues involved in RNA binding 50 Introduction 50 Materials and Methods 51 General materials 51 Cloning of E coli Hfq 53 Site-directed mutagenesis of Hfq 56 Over-express and purification of Hfq protein 59 HPLC-purification of the synthesized RNA oligomers 65 Sequence analysis of Hfq 68 Gel mobility shift assay 68 Fluorescence quenching study 69 Fluorescence anisotropy measurements 70 Results and Discussion 72 Sequence-structure analysis: identifying potential RNA 72 binding sites in Hfq Covariance of residue properties at spatially adjacent 77 positions vii The effect of Hfq mutations on RNA binding 78 Gel mobility shift assay of Hfq-RNA binding 79 Fluorescence anisotropy measurements of Hfq-A18 binding 87 Fluorescence quenching measurements 91 Summary 94 Appendix I 98 Appendix II 100 References 103 Chapter IV A structure transition involving dimerization of 79 nt 106 RNA Introduction 106 Materials and methods 107 Plasmid construction 107 In vitro transcription 108 Temperature gradient gel electrophoresis (TGGE) of RNA 109 Sedimentation equilibrium experiments 109 CD spectroscopy 110 RNase H assay 111 Hybridization Assay 111 Results and Discussion 112 Non denaturing PAGE studies of the RNA 112 TGGE studies of the RNA 115 Sedimentation equilibrium experiments 117 viii Predicated structure of 79 mer monomer and dimer 118 Circular dichroism spectra analysis 120 RNase H assay 124 Hybridization assay 126 References 130 VITA 131 ix LIST OF TABLES TABLE PAGE 2.1 Presence and absence of Hfq from BLAST search of bacterial 43 genomes 3.1 hfq gene PCR reaction 53 3.2 PCR reaction for site directed mutagenesis 58 3.3 Primers used fro site-directed mutagenesis PCR 58 3.4 HPLC program for separation of RNA 66 3.5 Reaction for radio-labeling DsrADII 69 3.6 Equilibrium dissociation constants of wild type and mutant Hfq 89 binding to A18 3.7 The saturated fluorescence quenching and stoichiometry of A18 for 93 Hfqs 4.1 RNA degradation by RNase H 125 x LIST OF FIGURES FIGURE PAGE 1.1 Model of secondary structure of 224 nt segments of 5’ leader region 4 of rpoS mRNA behind translation start points 1.2 Different known and potential regulatory outcomes brought about by 6 sRNA base-pairing with mRNAs 1.3 Model of Hfq facilitated pairing of domain I of DsrA with region 8 upstream of RBS of rpoS mRNA 1.4 Model for sodB mRNA−Hfq−RyhB interaction 9 1.5 Model for growth rate-regulated ompA mRNA translation and 11 stability by Hfq. 1.6 Mechanisms by which Hfq might facilitate sRNA-mRNA basepairing 16 2.1 Multiple alignment of Hfq proteins from 26 bacterial genomes 29 compared with the LSm protein from M.thermoautotrophicum and a consensus sequence for Sm proteins 2.2 3D line representations of the Hfq structure predicted by the 3D- 32 PSSM web server using the above archaeal Sm protein as template 2.3 The 3D structure of Hfq generated by SWISS-MODEL program 35 using the same archaeal LSm protein determined to be the best template by 3D PSSM 2.4 Molecular representation of the β4 and β5 strands in Hfq model 37 2.5 Unrooted neighbor-joining tree inferred by analysis of Hfq protein 44 sequences. Sequences were aligned using CLUSTAL program and all positions with gaps were excluded from the analysis 2.6 Conserved amino acid residues specific to Hfq proteins from major 45 bacterial groups defined by phylogenetic analysis 3.1 Thermal cycling of PCR reaction 54 3.2 pTYB11 plasmid and the multiple cloning site of pTYB11 55 xi 3.3 Overview of the QuickChange site-directed mutagenesis method 57 3.4 Thermal cycling of PCR reaction for site-directed mutagenesis 59 3.5 Schematic illustration of the IMPACT-CN System 61 3.6 SDS-PAGE of the fractions collected from each step in the Hfq 61 expression and purification process 3.7 SDS-PAGE of wild type and mutant Hfq proteins 63 3.8 UV spectrum of purified wild type Hfq protein 64 3.9 CD spectrum of purified wild type Hfq protein 64 3.10 The predicted 2nd structure of DsrA RNA 65 3.11 Schematic representation of the determination of receptor-ligand 71 interaction by fluorescence anisotropy 3.12 Multiple alignment of Hfq conserved domain from representative 74 bacterial genomes 3.13 Space-filling representations of E coli Hfq 75 3.14 The Hfq sequences which involve amino acid covariance in L12 and 77 F39 3.15 Gel shift experiments showing the binding of wild type and three 80 mutant Hfq proteins to OligoA18 RNA. 3.16 Quantification of the gel shift experiments of A18 for wild type and 81 all the mutant Hfqs. 3.17 Gel shift experiments showing the binding of wild type and three 83 mutant Hfq proteins to radio-labeled DsrADII RNA 3.18 Quantification of the gel shift experiments of DsrADII for wild type 84 and all the mutant Hfqs 3.19 Gel shift experiments showing the binding of wild type and three 85 mutant Hfq proteins to fluorescence labeled DsrADII RNA 3.20 Stoichiometry of Hfq:DsrADII complexes 86 xii 3.21 Fluoresence anisotropy data of wt Hfq and mutant Hfqs binding to 88 A18 3.22 Fluorescence anisotropy data of wt Hfq and mutant Hfqs binding to 90 DsrADII 3.23 Fluorescence Emission spectra and titration of wild type Hfq with 92 oligoA18 3.24 Multiple alignments of All Hfq proteins found in microbe 99 3.25 The multiple alignment of Sm/Lsm protein 101 3.26 The 3-D structure of A. fulgidus Sm1 102 4.1 Construction of the modified pGEM7Zf(+) 107 4.2 Refolding of the RNA molecules after heating assayed by 12% 113 PAGE in TBE buffer 4.3 Refolding of the RNA molecules assayed by 12% PAGE in TBM gel 114 buffer 4.4 Temperature Gradient Gel Electrophoresis of the RNA in TBE buffer 116 4.5 Temperature Gradient Gel Electrophoresis of the RNA in TBM 116 buffer 4.6 The predicted lowest free energy secondary structure of 79 nt RNA 119 monomer and dimer 4.7 AB CD spectra of monomer and
Details
-
File Typepdf
-
Upload Time-
-
Content LanguagesEnglish
-
Upload UserAnonymous/Not logged-in
-
File Pages149 Page
-
File Size-