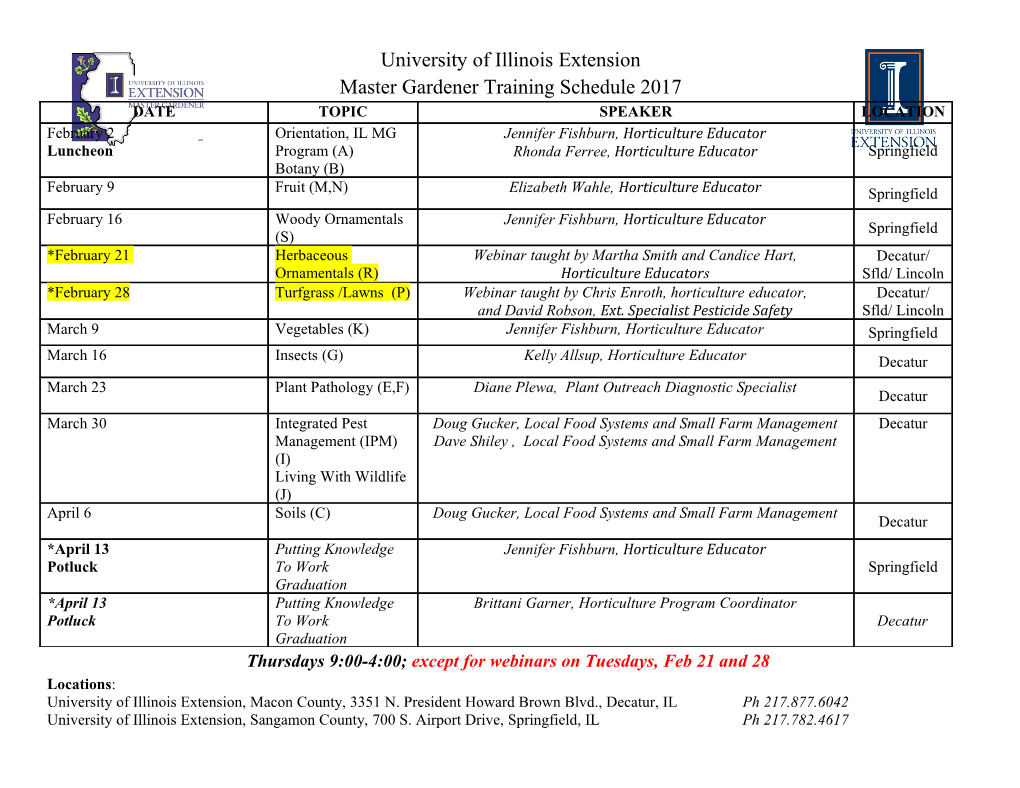
ISIJ International, Vol. 44 (2004), No. 6, pp. 999–1005 Reduction of Titania–Ferrous Ore by Hydrogen Eungyeul PARK and Oleg OSTROVSKI1) Former PhD Student, School of Materials Science and Engineering, University of New South Wales, Sydney 2052, Australia, now at Max-Planck-Institut für Eisenforschung, Max-Planck Str. 1, D-40237 Düsseldorf, Germany. E-mail: [email protected] 1) School of Materials Science and Engineering, University of New South Wales, Sydney 2052, Australia. (Received on November 4, 2003; accepted in final form on February 16 2004 ) The reduction of titania–ferrous ore (ironsand) containing 57.2 mass% of iron and 7–8 mass% of TiO2 was investigated in isothermal experiments using H2–Ar gas mixtures in a laboratory fixed bed reactor in the temperature range from 973 to 1 373 K. The degree of reduction was measured using an on-line Dew Point sensor and the samples in the course of reduction were characterized using SEM and XRD analyses. The complete reduction of iron oxide in the ore by 25vol%H2–Ar was achieved within 60 min at tempera- ture higher than 1 123 K. At 1 173 K, the reduction rate increased with hydrogen content in the reducing gas up to 25 vol%H2. The composition of samples after 2-h reduction by 25vol%H2–Ar depended on the reduc- tion temperature. Below 1 073 K, the final sample contained iron and iron–titanium oxides. At temperatures above 1 173 K, the final sample was composed of iron and titanium oxide. The reduction path at tempera- tures above 1 173 K is suggested as follows: → ϩ → ϩ → ϩ Ͻ Ͻ Ϫ Fe3ϪxTixO4 ‘FeO’ Fe3ϪxϪdTixϩdO4 Fe Fe3ϪxϪdTixϩdO4 Fe xTiO2,(0d 1 x) KEY WORDS: ironsand; titanimagnetite; reduction; hydrogen; reduction path. processes with carbon monoxide, it is necessary to clarify 1. Introduction the mechanism of the reduction of ironsand by hydrogen for Among titania–ferrous iron ores found throughout the the future application. world, the ironsand from New Zealand is in a large deposit This work presents results of reduction of ironsand by and contains about 57 mass% of total iron, with the price of hydrogen, discusses the reduction mechanism by the exami- about 2/3 of the conventional hematite iron ores.1–5) The nation of the phase transformation during the reduction, ironsand consists of homogeneous particles in majority, and compares with hematite and magnetite iron ores and and non-homogeneous particles. The homogeneous parti- previous data. cle is composed of titanomagnetite (TTM), a magnetite (Fe O )–ülvospinel (Fe TiO ) solid solution (Fe Ti O , 3 4 2 4 3Ϫx x 4 2. Experimental with xϭ0.27Ϯ0.02). The non-homogeneous particle is composed of titanohematite (TTH, Fe2ϪyTiyO3) phase and The chemical compositions of ironsand and hematite TTM phase.4,6,7) iron ore examined in this work are presented in Table 1. The application of ironsand for the direct reduced iron The particle size of ores was screened to be in the range of (DRI) process has been studied for many decades. It has 125–212 mm. The surface area was 1.2ϫ103 m2/kg for iron- been known that the reduction of ironsand is slower than of sand and 1.6ϫ103 m2/kg for hematite iron ore. The mass of hematite or magnetite iron ores.3,4,7–11) In the reduction of the ore in each experiment was 2 g. The oxygen associated ironsand by carbon monoxide, the complete reduction of with iron was calculated to be 21.1 mass%. The extent of iron oxides requires temperature above 1 273 K with high reduction was calculated as a mass fraction of oxygen in reducing potential. In the previous work by the authors,7) iron oxides removed in the course of reduction. the reason for the stability of the ironsand against carbon Reduction of ores using H2–Ar gas mixture was studied monoxide atmosphere was suggested to be the structure and in a laboratory fixed bed reactor in a vertical tube electric higher thermodynamic stability of TTM resulted from the existence of titanium. Table 1. Chemical compositions (mass%) of ironsand and While the mechanism of the reduction ironsand by car- hematite iron ore. bon monoxide has been studied intensively by a few re- search groups,4,7–10) the information on the reduction of ironsand by hydrogen is still not sufficient, especially in the kinetics and the phase transformation during the reduction. Because hydrogen is a major reducing agent in DRI 999 © 2004 ISIJ ISIJ International, Vol. 44 (2004), No. 6 Fig. 1. (a) Schematics of experimental set-up: 1 - reactor, 2 - pressure gauge, 3 - six way valve, 4 - rotameter, 5 - gas flow controller, 6 - gas purifier and 7 - heating tape. (b) Schematic experimental reactor. furnace. Experimental set-up and the schematic of the reac- tor are presented in Fig. 1. After a sample was put into the reactor, it was purged with argon. Then the reactor was moved to the hot zone of the furnace. A sample was heated to a required temperature in argon atmosphere. Then the re- ducing gas mixture was introduced to the reactor. After cer- tain reaction time, the reactor was pulled off from the hot zone of the furnace and quenched. The hydrogen and the argon used in the experiments were super high purity and high purity, respectively. The gases were purified before mixing by passing through traps filled with Drierite and 4 A molecular sieve to remove moisture. The composition of re- ducing gas was achieved using gas flow controllers (Brooks, Model 5850E). The outlet gas was analyzed on- line by a dew point sensor (General Eastern Hygro M4/D- Fig. 2. Reduction of ironsand by 25vol%H2–Ar gas mixture at 2). The outlet gas line from the top of the reactor to the dew different temperatures. point sensor was heated by a heating-tape with temperature about 393 K. The total gas flow rate was maintained at Ϫ 1 123 K. The degrees of reduction of the sample reduced at 1.33ϫ10 5 m3/s. 973 and 1 073 K after 2-h reduction were about 70 and Samples were analyzed with X-ray Diffractometer (XRD, 95%. The reduction rate increased with temperature from SIEMENS D5000). The XRD has a monochromator and a 973 to 1 273 K; further increase in temperature to 1 373 K copper Ka X-ray source. The voltage and current in the X- had a negligible effect. At 1 273 and 1 373 K, the reduction ray emission tube were set at 30 kV and 30 mA, respective- extent increased linearly with time up 80% of reduction ly. The scanning rate on samples was set at 0.6°/min with a and slowed down afterwards until the completion of the re- step of 0.03°. The morphology of samples was observed by duction. In the temperature range of 973 to 1 173 K, the Field Emission Scanning Electron Microscope (FESEM, slope of the reduction curves slowly and steady decreased, HITACHI S-4500). The extent of reduction was calculated with increasing extent of reduction. as a mass fraction of oxygen in iron oxides removed in the course of reduction. 3.2. Effect of Hydrogen Content Effect of hydrogen content on the reduction of ironsand 3. Results was studied by reaction of ironsand with H2–Ar gas mixture at 1 173 K. The hydrogen content was varied from 5 to 50 3.1. Effects of Temperature vol%. The reduction curves are presented in Fig. 3. Reduction of ironsand by hydrogen was studied using As shown in Fig. 3, increase in hydrogen content from 5 H2–Ar gas mixtures in the temperature range of 973 to to 10 vol% caused a sharp increase in the reduction rate. 1 373 K. Reduction curves are presented in Fig. 2. In the Reduction rate increased with increasing hydrogen content experimental range, iron oxides in the ironsand were re- up to 25 vol% H2; further increase to 50 vol% had a rela- duced to metallic iron completely at temperatures above tively slight effect. © 2004 ISIJ 1000 ISIJ International, Vol. 44 (2004), No. 6 3.3. Phase Transformation during the Reduction of iron became dominant in the XRD pattern, and some traces Ironsand by H2–Ar Gas Mixture of TTM peaks appeared. The reduction of TTM to wüstite Samples in the progress of reduction at 1 173 K by proceeded much slower than reduction of wüstite to metal- lic iron. Wüstite was reduced very rapidly, what explains 25vol%H2–Ar gas mixture were analysed by XRD as shown in Fig. 4. Metallic iron was observed after 3 min of the weakness of wüstite peaks in the XRD patterns, which reduction, when the reduction extent was about 15%. consistent with the results of the reduction by carbon Wüstite peaks were not detected except a trace in the XRD monoxide by the authors.7) In the XRD pattern of a sample pattern of a sample reduced for 5 min. After 20 min, when reduced for 60 min, when 100% of reduction of iron oxides the reduction extent achieved 80%, the peak for metallic was achieved, the peaks of Ti-containing phases; TTM, il- menite and Ti oxides, were not detected. Figure 5 presents XRD patterns of the samples reacted by 25vol%H2–Ar for 2 h at different temperatures from 973 to 1 373 K. Due the high intensity of the iron peak at around 45°, a part of the whole pattern from 25 to 40° was en- larged for the identification of minor phases. While there is no evidence of Ti-containing phases in the XRD patterns of the sample reduced at 1 173 K, those reduced at 973 and 1 073 K contained Fe–Ti oxides (TTM and ilmenite) and the samples reduced at 1 273 and 1 373 K contained Ti oxide phase.
Details
-
File Typepdf
-
Upload Time-
-
Content LanguagesEnglish
-
Upload UserAnonymous/Not logged-in
-
File Pages7 Page
-
File Size-