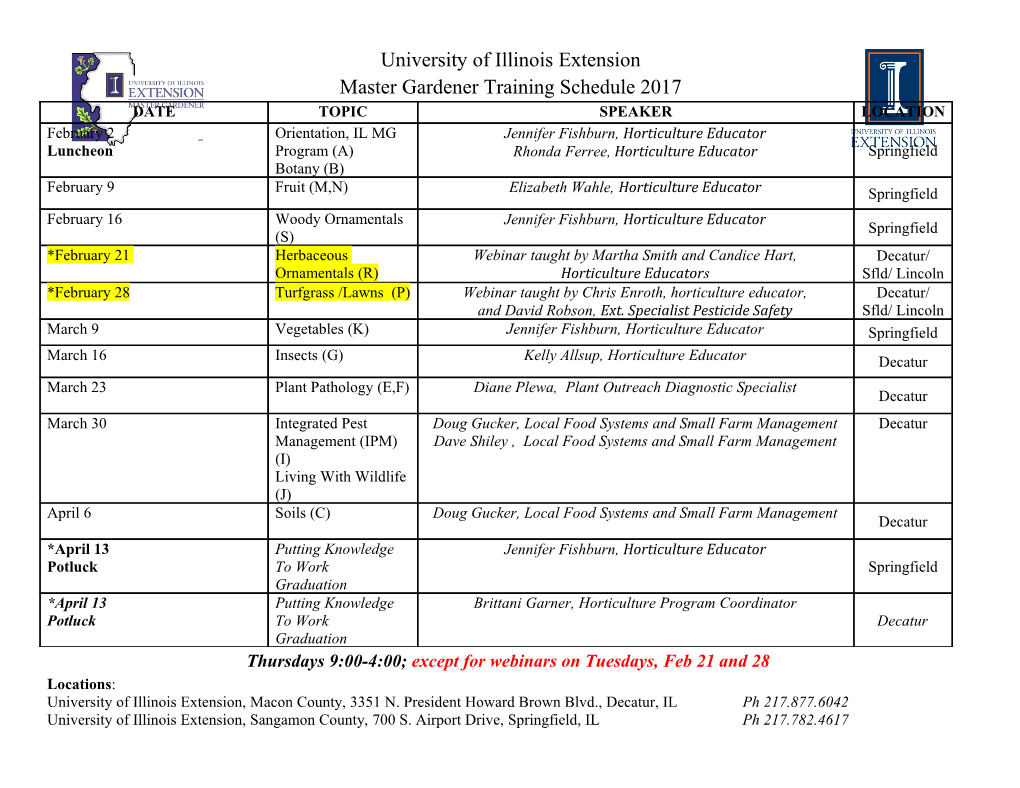
Published on Web 05/12/2006 Electrochemistry at Single-Walled Carbon Nanotubes: The Role of Band Structure and Quantum Capacitance Iddo Heller, Jing Kong,† Keith A. Williams,‡ Cees Dekker, and Serge G. Lemay* Contribution from the KaVli Institute of Nanoscience, Delft UniVersity of Technology, Lorentzweg 1, 2628 CJ Delft, The Netherlands Received February 20, 2006; E-mail: [email protected] Abstract: We present a theoretical description of the kinetics of electrochemical charge transfer at single- walled carbon nanotube (SWNT) electrodes, explicitly taking into account the SWNT electronic band structure. SWNTs have a distinct and low density of electronic states (DOS), as expressed by a small value of the quantum capacitance. We show that this greatly affects the alignment and occupation of electronic states in voltammetric experiments and thus the electrode kinetics. We model electrochemistry at metallic and semiconducting SWNTs as well as at graphene by applying the Gerischer-Marcus model of electron transfer kinetics. We predict that the semiconducting or metallic SWNT band structure and its distinct van Hove singularities can be resolved in voltammetry, in a manner analogous to scanning tunneling spectroscopy. Consequently, SWNTs of different atomic structure yield different rate constants due to structure-dependent variations in the DOS. Interestingly, the rate of charge transfer does not necessarily vanish in the band gap of a semiconducting SWNT, due to significant contributions from states which are a few kBT away from the Fermi level. The combination of a nanometer critical dimension and the distinct band structure makes SWNTs a model system for studying the effect of the electronic structure of the electrode on electrochemical charge transfer. Introduction demonstrated that semiconducting SWNTs can act as channels Carbon is widely used as electrode material for electrochem- in nanoscale transistors, allowing their conductance to be tuned 10 istry. The combination of a wide useful potential window, by electrostatic interaction with a solid-state gate or an 11 electronic properties similar to metals, and its versatile organic electrolyte gate. A potential applied between an SWNT and chemistry make sp2 carbon a special electrode material.1 the electrolyte it is immersed in can very effectively change Recently, there has been a large interest in using single-walled the chemical potential of the SWNT. Since this interfacial carbon nanotubes (SWNTs) as electrodes for electrochemistry.2-4 potential difference drives electrochemical reactions in voltam- This interest mostly originates from the prospect of using metric experiments, electrolyte gating and electrochemical individual SWNTs as carbon nanoelectrodes or ensembles of charge transfer are inevitably coupled. SWNTs as large surface-area carbon electrodes. The molecules’ In previous work, we demonstrated that individual SWNTs interesting, unconventional electronic properties, however, are can be used as nanoelectrodes for electrochemistry, yielding often neglected. Depending on their chirality, SWNTs behave enhanced mass transport and high current densities equivalent either as a metal or a semiconductor. They possess a distinct to sub-10 nm hemispherical electrodes.2 Due to their nanometer and nontrivial density of electronic states (DOS) displaying van critical dimension, electrode kinetics are accessible even for fast Hove singularities typical for one-dimensional conductors,5-7 electrode reactions such as the oxidation of ferrocenes. Since as has been observed in STM spectroscopy.8,9 It has been the kinetics of electrochemical charge transfer are affected by the occupation and alignment of electronic states in solution † Present address: Department of Electrical Engineering and Computer and on the electrode,12,13 it is important to understand in detail Science, Massachusetts Institute of Technology, 77 Massachusetts Avenue, Cambridge, MA 02139-4307. the effect of both electrolyte gating and a distinct electronic ‡ Present address: Department of Physics, University of Virginia, 382 structure on electrochemistry at SWNTs. McCormick Road, Charlottesville, VA 22904-4714. (1) McCreery, R. L. Electrochemical properties of carbon surfaces. ed.; In this paper, we introduce the relevant features of the Dekker: New York, 1999; p 631. electronic structure of SWNTs in the context of electrolyte (2) Heller, I.; Kong, J.; Heering, H. A.; Williams, K. A.; Lemay, S. G.; Dekker: C. Nano Lett. 2005, 5 (1), 137-142. (3) Gooding, J. J. Electrochim. Acta 2005, 50 (15), 3049-3060. (9) Odom, T. W.; Huang, J. L.; Kim, P.; Lieber, C. M. Nature 1998, 391 (6662), (4) Katz, E.; Willner, I. ChemPhysChem 2004, 5, 1048. 62-64. (5) Dekker, C. Physics Today 1999, 52 (5), 22-28. (10) Tans, S. J.; Verschueren, A. R. M.; Dekker, C. Nature 1998, 393 (6680), (6) Saito, R.; Fujita, M.; Dresselhaus, G.; Dresselhaus, M. S. Appl. Phys. Lett. 49-52. 1992, 60 (18), 2204-2206. (11) Rosenblatt, S.; Yaish, Y.; Park, J.; Gore, J.; Sazonova, V.; McEuen, P. L. (7) Dresselhaus, M. S.; Dresselhaus, G.; Saito, R. Solid State Commun. 1992, Nano Lett. 2002, 2 (8), 869-872. 84, 201. (12) Adams, D. M. et al. J. Phys. Chem. B 2003, 107, 6668. (8) Wildoer, J. W. G.; Venema, L. C.; Rinzler, A. G.; Smalley, R. E.; Dekker, (13) Bard, A. J.; Faulkner, L. R. Electrochemical Methods, Fundamentals and C. Nature 1998, 391 (6662), 59-62. Applications, 2nd ed.; John Wiley & Sons: New York, 2001. 10.1021/ja061212k CCC: $33.50 © 2006 American Chemical Society J. AM. CHEM. SOC. 2006, 128, 7353-7359 9 7353 ARTICLES Heller et al. Figure 1. Nearest-neighbor tight-binding calculation of the density of electronic states (DOS) as a function of energy for a graphene sheet (black), a metallic (9,0) SWNT (blue), and a semiconducting (10,0) SWNT (red). γ0 ) 2.7 eV is the nearest-neighbor interaction energy. gating. We combine electrochemical gating and electrochemical charge transfer by applying the Gerischer-Marcus model of heterogeneous electron transfer kinetics to SWNTs and show that the interfacial capacitances have a large impact on the behavior of electron transfer at SWNT electrodes. The potential- dependent rate of electron transfer reveals a rich spectrum, Figure 2. (a) Schematic representation of a measurement layout showing an SWNT submerged in an electrolyte. A potential Vappl is applied between caused by the distinct SWNT band structure, allowing for a the SWNT and a reference electrode in solution, inducing an electrical form of electrochemical spectroscopy to be performed. Due to double layer at the SWNT-electrolyte interface. (b) The interfacial atomic-structure-dependent variations of the features in the DOS, capacitance between SWNT and electrolyte can be represented as the we predict relatively large differences between electron transfer electrostatic double-layer capacitance Cdl in series with the chemical quantum capacitance Cq. Although they are represented as linear circuit rates at different SWNTs. We relate our calculations to classic elements here, both are in fact nonlinear: Cq ) Cq(Vch) and Cdl ) Cdl(Vdl). Butler-Volmer kinetics in an experimentally relevant regime (c) Energy diagram showing the influence of the quantum capacitance on and observe a clear diameter-dependent trend in the apparent the alignment and occupation of electronic states in the SWNT. The reference energy in this diagram is set by the reference electrode. In the standard rate constant. initial situation, the Fermi level is positioned in the valence band of the SWNT. In the classical case Cq . Cdl, application of a positive voltage SWNT Electronic Density of States (DOS) Vappl > 0 will shift the band structure by eVdl with respect to its initial position, while the position of the Fermi level with respect to the band Graphene consists of a two-dimensional hexagonal lattice of edges remains unchanged. In the extreme opposite case, Cq , Cdl, the sp2 carbon, through which electronic conduction can occur via position of the band gap remains unchanged with respect to the reference the π-conjugated electron system.14 Graphene is sometimes energy, while the Fermi level shifts by eVch with respect to the bands. classified as a zero-gap semiconductor, since the DOS per unit area, Fgraph(), vanishes at the Fermi level. (For clarity we will typical positions of van Hove singularities are different for use for energies, units eV, and V for voltages, units V.) The metallic and semiconducting SWNTs. At this level of ap- band structure of an SWNT can be described by considering proximation the DOS of SWNTs and graphene are symmetrical the nanotube as a graphene sheet wrapped into a cylinder. The around the half-filling energy, hf, defined as the energy at which atomic structure of an SWNT is conventionally described by all bonding orbitals are filled and all antibonding orbitals are two indices (n,m) that fully define the radius and chirality.5 The empty, yielding exactly one occupied π-orbital per carbon atom. (n,m) indices also determine the electronic structure of the Quantum Capacitance SWNT.6 If |n - m| ) 3q, where q is an integer, the SWNT is metallic, whereas for |n - m| * 3q it is semiconducting and a SWNTs can be employed in a field-effect-transistor layout band gap occurs in the DOS. The size of the band gap is with an electrolyte in contact with the SWNT acting as a gate,11 inversely proportional to the diameter, yielding band gaps in as illustrated in Figure 2a. Note that, to simplify the notation the range 0.3 to 0.8 eV for SWNTs with diameters of 3 to 1 when relating energy changes to potential changes, we use the nm. Figure 1 shows nearest-neighbor tight-binding calculations convention that potentials are applied to the reference electrode 14 of the π-electron DOS of a graphene sheet and of a metallic with respect to the working electrode. A potential Vappl applied and semiconducting SWNT.6,7 The SWNT DOS roughly follows between the SWNT and the electrolyte through a reference the graphene DOS but exhibits distinct singularities that are electrode in solution can cause a net charge to build up in the typical of one-dimensional electronic bands.
Details
-
File Typepdf
-
Upload Time-
-
Content LanguagesEnglish
-
Upload UserAnonymous/Not logged-in
-
File Pages7 Page
-
File Size-