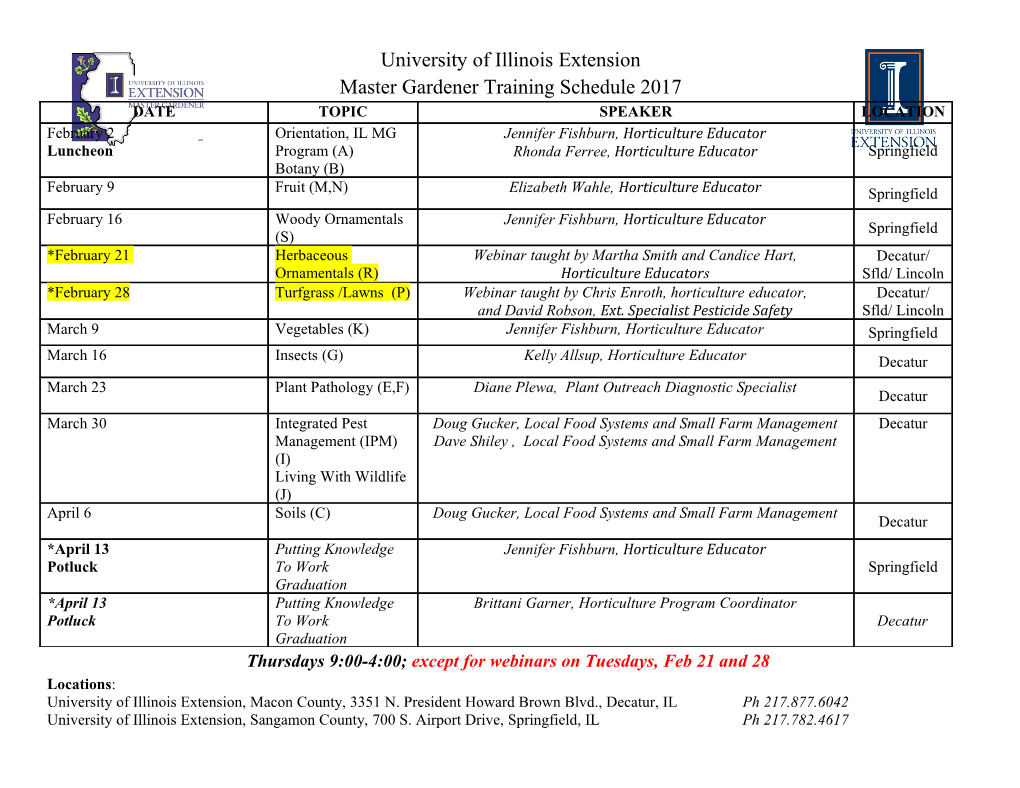
Cell Membrane Potential Resting Membrane Potential • Resting membrane potential: for quiescent cells The genesis of resting potential is mainly the result of • Action (membrane) potential: excited cells • The concentrations of diverse ions which prevail inside and outside of the cell close to the surface of the Both resting and action potentials are produced by temporary changes in the current flow across the membrane. membrane, namely, in the ionic flow through the resting and gated channel proteins in the membrane. • The selective permeability of the cell membrane to particular ions. Each and every biosignal has its origin in a brief imbalance of the resting membrane potential, irrespective of the biosignal’s type. Basic Biophysics Tools and Relationships Resting Potential of a Membrane Permeable to One Ion 11.4.1 Basic Laws J J (diffusion) J (drift) 0 -Fick’s Law: diffusion Einstein Relationship K kT [K ] d[I] D kT o E vi vo ln EK+: Nernst potential J D K q [K ] dx q i Same for Na+ and Cl- -Ohm’s Law: drift (: mobility, Z: ionic valence, [I] ion concentration) dVm Donnan Equilibrium J Z[I] - Suppose a membrane is permeable to both K+ and Cl-. dx [K ]o [Cl ]i E E K Cl [K ]i [Cl ]o Goldman Equation - The Goldman equation describes the relationship between Vm and permeable ions. - Valid when the membrane potential or electric field is constant. (good for resting potential) qV V KKe kT K oi J qV K kT e 1 qV V Na Na e kT Na o i J qV Na kT e 1 qV V Cl ekT Cl Na o i J qV Cl kT e 1 Goldman Equation - Space-charge neutrality: J J J K Na Cl kT P [K ] P [Na ] P [Cl ] V ln K o Na o Cl i m q PK [K ]i PNa[Na ]i PCl [Cl ]o kT D P , P , P Permeabilities, eg. P K K K Na Cl K q : membrane width Ion Pumps - Assuming an active K+ pump, passive channels for K+ and Cl- dK[] dv JJ D ZK[] K pump Kdx K K dx dCl[] dv JD ZCl[] Cl Cldx Cl Cl dx 2kT J K [K ] [K ] pump q o i Membrane Potentials Resting Membrane Potential - Selective permeability of the membrane to ions: membrane potential - For neurons, 60 ~ 90 mV Significant ions determining the resting potential are K+, Na+, and Cl- ions: K+ ions and large immobile anions - By convention, V(out) = 0; the resting potential Vm = V(in) – V(out) = -60 mV. prevail in the intracellular space while Na+ and Cl- ions prevail in the extracellular space. Active mechanisms - Hyperpolarization: Vm < -60 mV are required to maintain the above concentration gradients across the membrane; for instance, the gradients of K+ and Na+ ions are due to the active sodium–potassium pump. - Depolarization: Vm > -60 mV • Inward electrical driving force on the permeable K+ ions, since these ions carry a positive electric charge. This electrical force opposes the •Outward chemical driving force (diffusional force) and thus limits the diffusional efflux of K+ ions. Graded Response An equilibrium is attained when the diffusional force driving K+ out of the cell is balanced by the electrical ∆Vm at the post-synaptic membrane force driving K+ into the cell. The Nernst equation gives - Due to a transformation from neurotransmitter chemical energy to electrical energy - Varies with the amount of neurotransmitter received The membrane is also slightly permeable to other ions such as Na+ and Cl- through (resting, nongated) - Sum or integration + - channel proteins in the membrane: UNa = 61 mV for Na ions and UCl = -68 mV for Cl ions. UK, UNa, UCl indicate the net driving force on the particular ion type. The Goldman–Hodgkin–Katz equation predicts the equilibrium voltage U with contributions of each ionic species. Resting Potential, Ionic Concentrations, and Channels - Resting potential: approximately -70 mV (the negative sign signifies excess negative Resting Membrane Potential charge inside the cell relative to the outside) • The factor pk quantifies the respective - Associated with ion transport and selective permeability of the membrane to ions permeability of the membrane to the k-th - Extracellular fluid: primarily Na+ and Cl- ion type; i.e., the ease with which the k-th + - ion type crosses the membrane. The typical - Cytoplasm: K and A (amino acids and proteins). ratios of permeabilities for the resting state - Ions pass through a channel. have been determined, being especially - Passive channels: always open and ion-specific (Na+, K+, Ca2+, and Cl-). applicable for the squid axon, as - Active channels: open in response to neurotransmitters and an appropriate change in membrane potential • U ~ -70 mV. The resulting electric field in the thin membrane of the cell (with the field magnitude U/d) is very high. The level of U determines the electrical force while UK, UNa, and UCl determine the respective electrochemical diffusional force. • A potential imbalance of the involved ionic concentrations in the cell, e.g., due to a slow depletion of ional gradients across the cell membrane in the course of the steady state, is prohibited by diverse active transport mechanisms in the membrane. These mechanisms maintain the ional gradients: K+ and Cl- ions are pumped back into the cell while Na+ ions back out of the cell. Equivalent Circuit Model for the Cell Membrane Equivalent Circuit Model for the Cell Membrane Equivalent Circuit Model for the Cell Membrane Neurons in Bioelectric Phenomena Introduction - Neurons - Hodgkin and Huxley model History - Galvani’s experiments with frog’s legs: action potential Neurons - nerve cells - neuroglial cells (10-50 times more, support) - cell body, dendrites (receiver), axon, and pre-synaptic terminals (transmitter) myelin sheath node of Ranvier (signal jump) -Signal velocity in the axon: 0.5 ~ 120 m/s http://stemcells.nih.gov/info/scireport/chapter8.asp GFP-tagged protein Dissociated culture in a rat hippocampal of rat hippocampal neuron neurons Université Laval, Canada Université Laval, Canada Dissociated culture of rat hippocampal neurons Université Laval, Canada Neurons • Numerous short cellular extensions, known as dendrites, which branch out in a tree-like fashion and provide a receptive area. The receptive area serves as a cellular input for graded inputs from other neurons (over neuronal synapses), from a physical stimulus (direct impact), or even from a nonneuronal receptor cell (over synapses). A membrane imbalance, known as the (electric) graded potential, is generated in this receptive area in response to any of the cellular inputs. •The cell body, known as soma, serves primarily as the metabolic center of the cell. • A single long tubular extension (from 0.1mm up to 3 m in length), known as the axon, conducts nongraded action potentials (all-or-none events). • At the end of the axon, a presynaptic terminal resides which releases a chemical transmitter in a graded manner (though in quantal steps). The transmitter release serves as a cellular output toward another neuron or a muscle cell. Types of neurons: • Sensory neurons, i.e., afferent neurons, include (or are interconnected with) sensory receptors located in the body or body’s periphery. The sensory neurons convey sensory information toward the central nervous system. Namely, sensory inputs such as light, sound, pressure, chemicals, or heat activate the corresponding receptors on the cellular level, e.g., activate gated channels for ions in the membrane of the dendrites. The resulting graded output of a receptor is converted into all-or-none action potentials which then propagate along the axon toward the neuronal synapses (as communication units with other neurons). Arriving in the central nervous system, an appropriate response is provoked, e.g., as a conscious perception or an involuntary reflex action. • Association neurons (interneurons) interconnect other neurons via synapses and ensure functional integrity of the central nervous system; These neurons comprise by far the largest class of neurons. • Motor neurons, i.e., efferent neurons, conduct action potentials from the central nervous system toward effector organs as muscle cells or glands. Namely, series of action potentials are converted into a graded release of a neurotransmitter (acetylcholine) in the neuromuscular synapses that triggers muscular contraction. Neurons • Neurons build specific (but not random) synaptic connections to other neurons, forming densely interconnected networks for functional processing, e.g., of incoming sensory information from the sensory neurons. The particular function of an embedded neuron in the network is greatly determined by its anatomical relationships to other neurons. On the input side, the neuron receives inputs either from sensory receptors (residing in the same neuron or in a receptor cell synaptically connected to this neuron, see below) or from other neurons (up to many thousands) via neuronal synapses located on dendrites and the cell body. On the output side, the axon branches out and builds collaterals (up to thousand) with the respective synapses in their terminal regions. These collaterals output a train of action potentials from the relevant neuron to numerous other neurons. In fact, the neuron is confronted with the task of decision- making based on prevailing inputs, to fire or not to fire action potentials on the output side—the very task of the nervous system. Two types of receptors cells • Primary receptors: specialized sensory neuron which exposes specific membrane regions in its bare dendrites. The free sensorial endings respond to a particular external stimulus by a corresponding graded change. The stimuli can be of thermal, mechanical, or chemical origin. Primary receptors include olfactory sensory neurons for the sense of smell. • Secondary receptors: separate nonneuronal receptor cell which is synaptically connected to an afferent sensory neuron. The receptor cell is usually highly specialized and converts the sensory stimulus (e.g., of acoustical, optical, or gustatory origin) into a graded change in its membrane potential, i.e., into its receptor potential.
Details
-
File Typepdf
-
Upload Time-
-
Content LanguagesEnglish
-
Upload UserAnonymous/Not logged-in
-
File Pages16 Page
-
File Size-