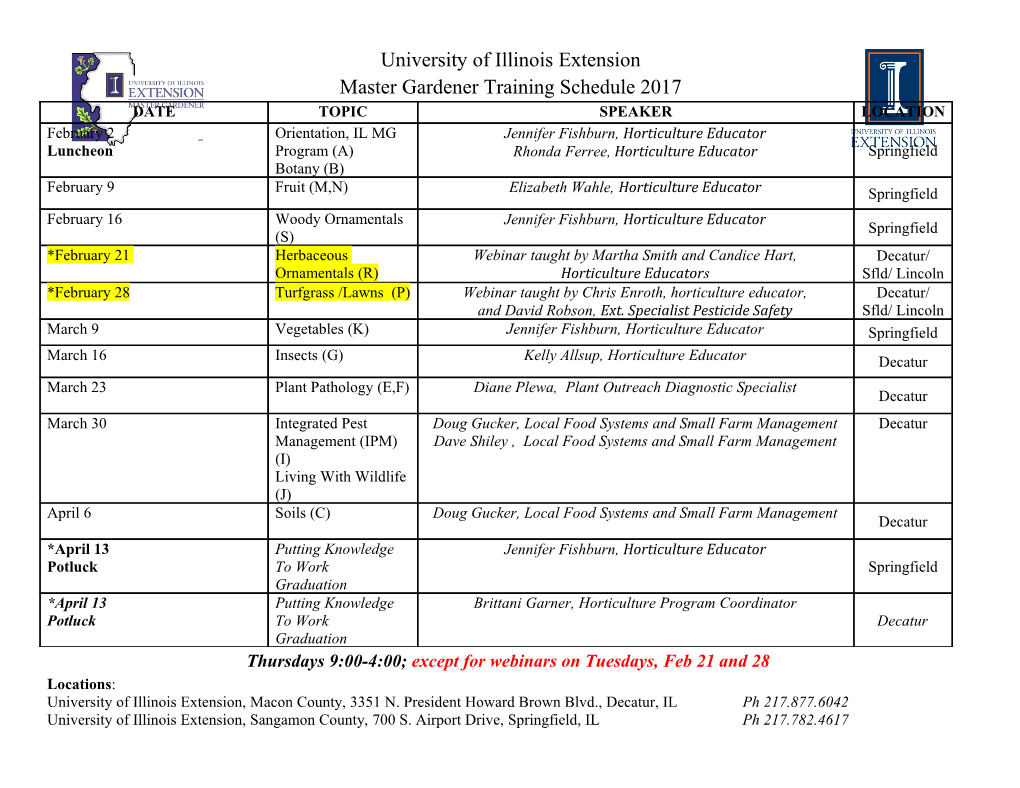
Chapter 22 Marieb & Hoehn (2019), p. 8181 We won’t do much anatomy here (left column; 22.1-22.3). Main issues (in lecture and lab): mechanics and measurement of breathing (22.4-22.5), chemoreceptor control of ventilation (22.8), and a few respiratory disorders (22.10). *** Sections 22.6-22.7 were briefly covered in Chapter 17. 1 Ch. 22: Test Question Templates • Q1. If given an appropriate graph of volume of air in lung vs. time, estimate or calculate FEV1/FVC ratio, FVC, residual volume, TLC, tidal volume, and/or minute ventilation. • Example (from Winter 2019 exam): 2 Q1. There are multiple ways to do this. The key is to choose a respiratory rate (breaths per minute) and a tidal volume (milliliters of air per breath) that, when multiplied together, give you 4000 mL air/minute. For example, I can draw a curve to show a tidal volume of 400 mL and a respiratory rate of 10 breaths/minute. Minute ventilation = (tidal volume)*(respiratory rate) = (400 mL air/breath)*(10 breaths/min) = 4000 mL air/min. 2 • Q2. If given two of the three values, calculate the third one: minute ventilation, respiratory rate, and tidal volume. • Example: Lola takes 10 breaths per minute, and her minute ventilation is 6000 mL air per minute. What is her tidal volume? • Q3. If given spirometry data and reference values, determine whether the data are consistent with obstructive pulmonary disease, restrictive pulmonary disease, both, or neither. • Example [from Fall 2019 test]: Rik is put through various pulmonary function tests. At time 0 in the table below, he begins deflating his fully inflated lungs as forcefully and rapidly as he can. You can assume that by the time of 4 seconds, he has reached his residual volume (RV). Based on these data, is he likely to have an obstructive lung disease? Explain your reasoning, citing any relevant math. 3 Q2. MV = TV*RR. Here, 6000 mL/min = TV*(10 breaths/min). TV = 600 mL/breath. Q3. A FEV1/FVC ratio of less than 0.8 (or 80%) suggests possible obstructive disease. Here FEV1 = 4.5 minus 3.0 = 1.5 Liters, and FVC = 4.5 minus 1.5 = 3.0 Liters, so FEV1/FVC = 1.5/3.0 = 0.5, or 50%. This low ratio is consistent with obstructive pulmonary disease. 3 • Q4. Given changes to components of Fick’s Law of Diffusion, say whether the diffusion rate will increase or decrease, or whether the information given is insufficient to determine this. • Example: The conditions in a patient’s lungs change such that the O2 concentration gradient from lungs to pulmonary capillary blood increases, and the diffusion barrier thickness also increases. What will be the net effect of these two changes on O2 diffusion rate into the blood? Explain the direction of change (increase or decrease), or explain why you can’t tell from the information given. 4 Q4. Example. According to Fick’s Law, a higher concentration gradient will increase the diffusion rate, but a thicker diffusion barrier will decrease the diffusion rate. Since these two changes have opposite effects on the diffusion rate, and since we don’t know the magnitude of either change (and thus whether one would “dominate” the other), the information given is not enough to establish whether diffusion rate will increase or decrease or neither. 4 Functions of the Respiratory System • 2 main ones • Bulk flow in and out of lungs (Poiseuille) • Diffusion of O2 and CO2 (Fick) S. Freeman et al. (2014), Figure 45.1 (like Marieb & Hoehn, Figure 22.1) 5 2 functions (bulk flow and diffusion, both of which reflect flow down a gradient (pressure and concentration, respectively). We’ll spend more time here on bulk flow. You already understand that bulk flow depends on a pressure gradient, but understanding how that works in the lungs is not trivial. *** A common misconception: if CO2 leaves the lungs, that “leaves more room” for O2 and vice versa. The term “gas exchange” is partly to blame. Each gas actually diffuses according to its own concentration gradient. *** Additional respiratory system functions: communication (voice), olfaction, innate immunity/protection from environment…. 5 The most important muscles for breathing Q1. What are they? Q2. Do they contract during inhalation or exhalation? Q3. How does their contraction change the thoracic cavity? Q4. How does their relaxation change the thoracic cavity? Marieb & Hoehn (2019), Figure 22.16 6 Respiratory muscles (just the most important ones). *** Q1. Diaphragm and external intercostal (“between-the-ribs”) muscles. Q2. Inhalation. Q3. When these muscles contract, the thoracic cavity increases in volume. Q4. When these muscles relax, the thoracic cavity decreases in volume. *** External intercostals are more lateral, internal intercostals are more medial. Both are pictured here. Internal intercostals can contribute to forced exhalation. 6 Why does air move in and out of the lungs? A simple description of breathing is this: The contraction of your diaphragm and external intercostal muscles expands your thorax. Since your lungs are tethered to the thorax walls, they expand too, and additional air enters the now-enlarged lungs. Then your inspiratory muscles relax and the process reverses. This description is true, but incomplete. It doesn’t explain scenarios such as pneumothorax, when a hole in the chest wall impairs breathing even if the lungs themselves are still intact. The thorax can still expand and shrink – so why don’t the lungs follow along any more? Clearly, the pressures in the different compartments must be important. For a fluid (air) to flow in bulk, there must be a pressure gradient. Our respiratory system manipulates volumes to create the desired pressure gradients and thus the desired flow (see following slides). 7 7 Changing a compartment’s pressure by changing its volume Boyle’s Law states that for a given quantity of gas molecules, the pressure and volume are inversely proportional -- as one goes up, the other goes down. Thus, to decrease the pressure of a compartment, increase its volume. To increase the pressure of a compartment, decrease its volume. Martini et al. (2015), Figure 23.12 8 This may sound like obscure chemistry, but we’ve actually encountered the general concept during our CV chapters! Heart chamber contracts => pressure in that chamber increases; chamber relaxes, pressure falls. Similarly, vessel vasoconstriction increases pressure and vasodilation decreases pressure. 8 The lungs are often represented as a balloon in a jar. Balloon = lungs, jar = thorax wall, space between them = pleural cavity. Contract inspiratory muscles, ↑ pleural cavity volume, ↓ pleural cavity pressure, ↑ transpulmonary pressure gradient, lung inflates Relax inspiratory muscles, ↓ pleural cavity volume, ↑ pleural cavity pressure, ↓transpulmonary pressure gradient, Freeman et al. (2017), Figure 44.12 lung deflates 9 9 Questions regarding the previous slide… Q1. Would the balloon still inflate if there was a hole in it? Q2. Would the balloon still inflate if there was a hole in the side of the jar? One more point…. Why doesn’t the balloon ever expand all the way to the sides of the jar? There must be an opposing force! Thus, the actual volume of normal lungs at any given time reflects the balance of 2 opposing forces… Finally, note differences between the balloon model and actual lungs. 1. The two lungs are in separate pleural cavities. 2. Our actual pleural cavity volume is TINY. 3. Diaphragm shapes/changes are different. 10 Q1-Q2. No. A (reasonably large) hole in either place would make it impossible to have a pressure difference between the inside of the balloon/lung and the outside of the balloon/lung. *** Opposing force: elastic recoil of balloon material (doesn’t “like” to be overstretched – just like the actual lungs). Thus, 2 opposing forces are: pressure gradient (drives lungs toward inflation) and elastic recoil (drives lungs toward deflation). Expansions of the lungs are opposed by their tendency to recoil elastically, so the lungs will never expand enough to eliminate the pressure gradient between the inside and outside of the lungs. *** Similarities of balloon model to actual lungs: basic principle of expand the volume around the lungs => decrease the pressure around the lungs => lungs inflate based on increased pressure gradient overcoming elastic recoil. Also, inhaling is the active process (requires muscle contraction). 10 Pneumothorax Q1. What does the word “pneumothorax” mean, based on its roots? Q2. A knife blade goes through the skin into the left pleural cavity, but does not touch the lungs. Which lungs will collapse, if any? youtube.com/watch?v=0vZ9gVyWreo 11 Q1. Pneumothorax means air in the thorax – specifically the pleural cavity. Q2. The left lung will collapse because the transpulmonary pressure gradient has collapsed. The right lung will not collapse because it resides in its own separate pleural cavity. *** X-ray of pneumothorax…. Note that the right side is “cloudy” while most of the left side is clear and dark. The “cloudiness” reflects the blood vessels, which do not stretch out across the thorax if the lung is collapsed. So the left lung is collapsed here. 11 Quantifying ventilation Q1. Definition of tidal volume? Q2. Definition of residual volume? Q3. Definition of Vital Capacity (VC or FVC)? What is it in this particular example? Marieb & Hoehn (2019), Figure 22.19 12 So far, we’ve focused on explaining why air moves in and out of lungs. NOW, let’s consider HOW MUCH air is moving in and out. Can make a graph… And name/label lots of quantities, of which we will focus on a few. *** Q1. Tidal volume = how much air goes in and out during one breath. Q2. Residual volume is the air that you can’t get out of your pulmonary system (lungs + conducting airways), no matter how hard you try.
Details
-
File Typepdf
-
Upload Time-
-
Content LanguagesEnglish
-
Upload UserAnonymous/Not logged-in
-
File Pages22 Page
-
File Size-