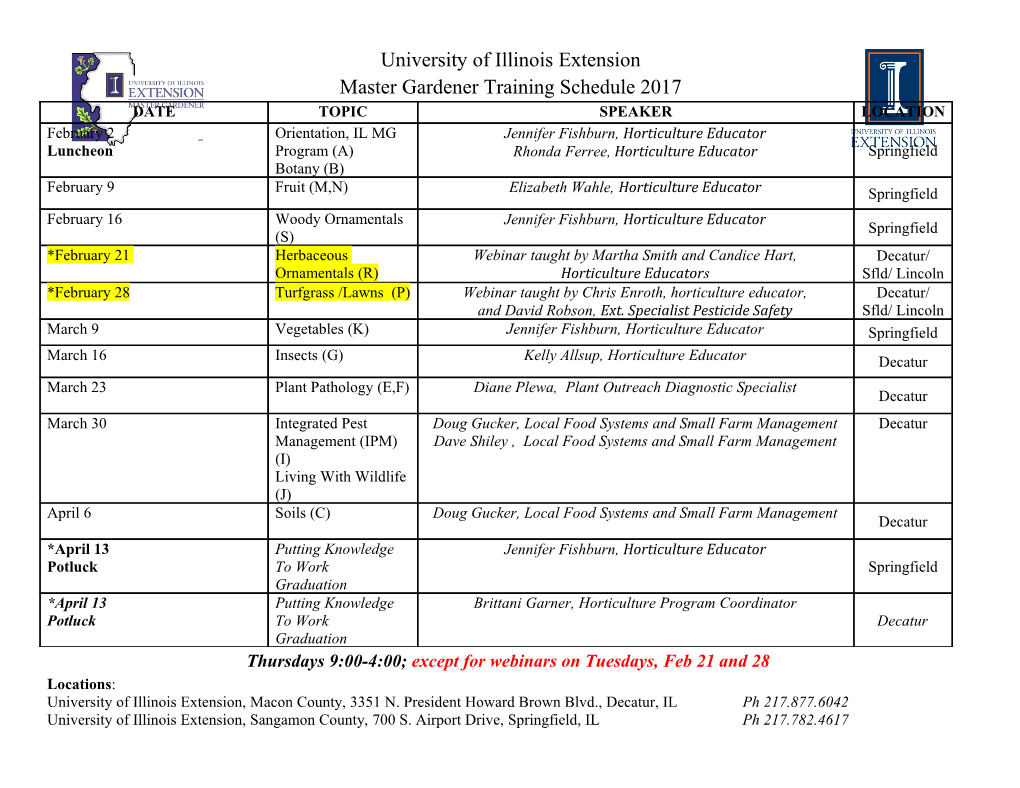
University of Groningen Magnesium and zinc hydride complexes Intemann, Julia IMPORTANT NOTE: You are advised to consult the publisher's version (publisher's PDF) if you wish to cite from it. Please check the document version below. Document Version Publisher's PDF, also known as Version of record Publication date: 2014 Link to publication in University of Groningen/UMCG research database Citation for published version (APA): Intemann, J. (2014). Magnesium and zinc hydride complexes: From fundamental investigations to potential applications in hydrogen storage and catalysis. [S.n.]. Copyright Other than for strictly personal use, it is not permitted to download or to forward/distribute the text or part of it without the consent of the author(s) and/or copyright holder(s), unless the work is under an open content license (like Creative Commons). The publication may also be distributed here under the terms of Article 25fa of the Dutch Copyright Act, indicated by the “Taverne” license. More information can be found on the University of Groningen website: https://www.rug.nl/library/open-access/self-archiving-pure/taverne- amendment. Take-down policy If you believe that this document breaches copyright please contact us providing details, and we will remove access to the work immediately and investigate your claim. Downloaded from the University of Groningen/UMCG research database (Pure): http://www.rug.nl/research/portal. For technical reasons the number of authors shown on this cover page is limited to 10 maximum. Download date: 02-10-2021 Reactivity studies Chapter 4 Reactivity studies This chapter contains investigations on the reactivity of the novel magnesium hydride clusters. The results are subsequently compared to the reactivity of zinc and calcium hydride complexes. Based on the prior observations a calcium-catalyzed hydrosilylation as well as a magnesium-catalyzed hydroboration is presented and potential catalytic cycles are proposed. Parts of this chapter will be submitted for publication: J. Intemann, S. Harder, manuscript in preparation. J. Intemann, S. Harder, manuscript in preparation. 125 Chapter 4 4.1. Introduction The strongly polar character of the M-H bond in main-group metal hydrides makes them a real or latent source of hydride anions which are one of the most powerful bases and known reducing agents. The heterolytic cleavage of the M-H bond can be brought about in three different ways: through nucleophilic substitution, oxidative addition of the hydride to an unsaturated substrate and protonolysis. This is the basis for the reducing, metalating, activating or drying properties for which main-group metal hydrides are well known.[1] Alanes, particularly LiAlH4, are widely used as powerful and to a greater or lesser extent selective reducing agents in organic as well as inorganic synthetic chemistry.[2] However, zinc hydride complexes of the type M[R2ZnH] (M = alkali metal, R = organic group) present a convenient alternative as they are powerful yet very selective reagents for the reduction of carbonyl groups in aldehydes and ketones as well as esters and amides.[3] Metal hydride complexes, and in particular transition metal hydride complexes, are valuable homogeneous catalysts. These form key intermediates in hydrogenation catalysis. A crucial feature to the reactivity of metal hydride complexes is the solubility of the reagent. On account of low solubility, calcium and magnesium dihydride are considered largely inert except for their reaction with water, which is very slow in the case of magnesium dihydride. For this reason magnesium dihydride is not even considered a drying agent, in contrast to calcium dihydride. The reactivity of a reagent can also be strongly influenced by the way it is prepared. Compact magnesium dihydride obtained from the uncatalyzed reaction of the elements with [4] each other is an essentially air-stable compound whereas finely divided MgH2 isolated from pyrolysis of diethyl magnesium reacts violently with water and ignites in air.[5] In 1978, Ashby et al. were able to show that a slurry of active MgH2 can be prepared by reaction of diethyl- or diphenylmagnesium with lithium aluminium hydride in diethylether. After separation of the ether soluble LiAlH2R2; THF has to be added to the ether wet MgH2 to obtain an active slurry.[6] Active MgH2 slurries were found to be capable of the reduction of several functional groups,[7] most noteworthy, the stereoselective reduction of cyclic and bicyclic ketones.[8] In addition, active MgH2 did not only dissolve in but also reacted with pyridine to form a mixture of 1,2- and 1,4-dihydropyridide (DHP) magnesium complexes. The 1,4-DHP magnesium complex was found to be the thermodynamically favored product and after four hours at 60 °C pure 1,4-DHP was isolated (Figure 4.1). The intermediate 1,2- dihydropyridide magnesium complex could not be isolated purely.[9] A similar observation was made for ZnH2 which also reacted with pyridine to form pure 1,4-dihydropyridide zinc 126 Reactivity studies complexes (Figure 4.1). In this case, the formation of 1,2-DHP zinc complexes was excluded on account of a D-labeling study: reaction of ZnH2 with pyridine-d5 gives exclusively products with H in 4-position.[10] Figure 4.1 Reaction of Mg and Zn hydride with an excess of pyridine. The formed bis(1,4-dihydropyridide) metal complexes could be successfully applied in the selective reduction of ketones, aldehyde, enones, imines and nitrogen containing heterocyclic compounds.[11,12] 1,4-Dihydropyridine is a crucial building block in the coenzyme nicotinamide adenine dinucleotide (NADH) and is responsible for reduction processes in biological systems. Therefore the synthesis of pure 1,4-dihydropyridine is important for the design of NADH-mimics. Alternative sources for 1,4-dihydropyridine moieties are e.g. Hantzsch esters.[13] Recently, Hill et al. demonstrated the dearomatization of pyridine and its derivatives with DIPP-Mg(n-Bu) and phenylsilane under formation of 1,2- and 1,4 dihydropyridide ß- diketiminate magnesium complexes (Figure 4.2). The 1,2-dihydropyridide magnesium complex was determined to be the kinetic product, while the corresponding 1,4- dihydropyridide complex is the thermodynamic one (heating the reaction mixture to 60 °C resulted in complete conversion to 1,4-DHP).[14,15] 127 Chapter 4 Figure 4.2 Dearomatization of pyridine by DIPP-Mg(n-Bu) and PhSiH3. The dearomatized pyridine derivatives are interesting substrates for homogenous catalytic transformations, however, reports are scarce. Those are limited to an initial report of a titanocene-based pyridine hydrosilylation by Harrod et al.,[16,17] followed by the description of a ruthenium-catalyzed process by Nikonov et al..[18,19] Recently, it has been shown that the organomagnesium complex, DIPP-Mg(n-Bu), is able to catalyze the hydroboration of pyridine and pyridine derivatives. In most cases preference for the 1,4-dihydropyridine product was observed but in other cases mixtures were found (Figure 4.3).[20] Figure 4.3 Magnesium-catalyzed hydroboration of pyridines. [21] The dearomatization of pyridine was achieved when using bis(allyl)calcium Ca(C3H5)2, herein the reaction of pyridine with the polar allyl calcium bond resulted in the regioselective 1,4-insertion. However, when methyl groups in ortho- or para-positions were present, metalation of the methyl groups was preferred over dearomatization of the pyridine ring.[22] A comparable 1,4-selective insertion of pyridine was also observed for the corresponding 1 1 alkali metal zincate [K(18-crown-6)][Zn(η -C3H5)3] and magnesate K2[Mg(η -C3H5)4]. In these cases the reaction was even shown to be reversible (Figure 4.4).[23] Figure 4.4 Schematic representation of the dearomatization of pyridine by metal allyl complexes. 128 Reactivity studies A further example of enhanced reactivity by increasing the solubility is the first soluble [24] calcium hydride complex [DIPP-CaH·THF]2 introduced by Harder et al.. Whereas solid CaH2 hardly reacts with any organic functional group and is therefore primarily used as drying agent for organic solvents, the soluble calcium hydride shows smooth reactivity for a [25] large scope of substrates (Figure 4.5). Additionally, [DIPP-CaH·THF]2 could also be [26] applied in the alkene hydrogenation with molecular H2 (20 bar). The calcium hydride complex was applied for the hydrosilylation of ketones, however, a detailed study of each step of the proposed catalytic cycle indicated that homoleptic R2Ca species are more efficient catalysts than heteroleptic LCaR complexes.[27] Figure 4.5 Reactions of the [DIPP-CaH·THF]2 complex (for simplicity represented as a monomer). Metal hydrides are also valuable precursors for the synthesis of metal amidoboranes from ammonia borane. For example, Ca(NH2BH3)2 can be synthesized by ball-milling of CaH2 and NH3BH3 at room temperature (Figure 4.6). 129 Chapter 4 Figure 4.6 Synthesis of Ca(NH2BH3)2 by ball-milling of CaH2 and NH3BH3. As mentioned before, metal hydrides are known to react with water forming the respective metal hydroxides. In a similar fashion, well-defined main-group metal hydroxide complexes could be obtained by the controlled hydrolysis of appropriate precursors. However, organometallic hydroxide complexes of group 2 elements are still scarce. This is due to the relatively weak ionic M-OH bond which results in fast ligand exchange and formation of insoluble M(OH)2. Four examples of magnesium hydroxide complexes have been reported to date. The first Ar,Me well-defined Mg-OH complex [Tp Mg(µ-OH)]2 (Tp = tris(pyrazolyl)hydroborate, Ar = p-(t- Bu)C6H4) was prepared by Parkin et al. by hydrolysis of the according magnesium methyl [28] precursor. Another magnesium hydroxide complex [DIPP-Mg(µ-OH)]2 was discovered by unintentional hydrolysis of the DIPP magnesium allyl complex and later reproduced by the controlled hydrolysis at low temperature of a corresponding DIPP magnesium amide.[29,30] The analogous [DIPP-Ca(µ-OH)]2 and [DIPP-Sr(µ-OH)]2 complexes were prepared via the same route.[31] Recently, a novel magnesium hydroxide cubane encapsulated by a polypyrrolic Schiff base macrocycle L (L2-[Mg4(µ-OH)4(OH)4]) has been reported.
Details
-
File Typepdf
-
Upload Time-
-
Content LanguagesEnglish
-
Upload UserAnonymous/Not logged-in
-
File Pages55 Page
-
File Size-