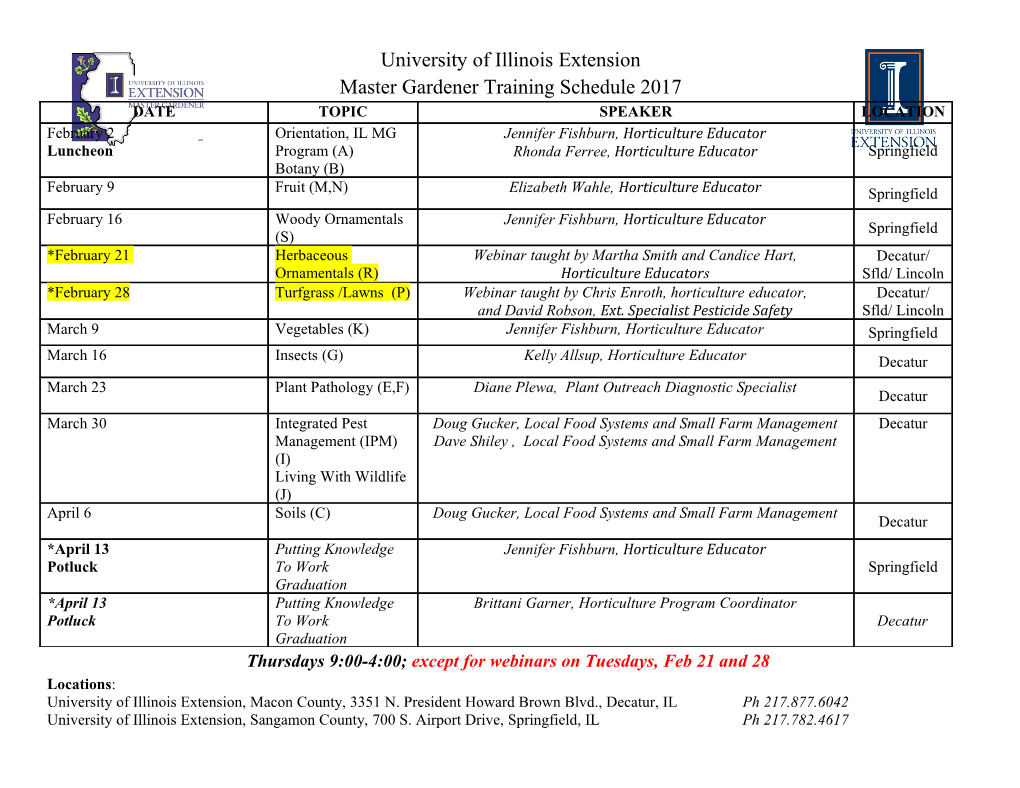
1 DELIVERY OF ANIMAL VIRUS DNA INTO THE NUCLEUS Urs F. Greber Institute of Zoology University of Zürich Winterthurerstrasse 190 CH-8057 Zürich Switzerland Phone: 41 1 635 4841, Fax: 41 1 635 6817, email: [email protected] 1. INTRODUCTION 2. CELL SURFACE BINDING AND UPTAKE Non-enveloped viruses 2.1. Adenoviridae: Adenovirus type 2 and type 5 (Ad-2 and Ad-5) 2.2. Papovaviridae: Polyoma virus and Simian virus 40 (SV40) 2.3. Parvoviridae: Parvovirus and Adeno-associated virus (AAV) Enveloped viruses 2.4. Herpesviridae: Herpes simplex virus type 1 (HSV-1) 2.5. Hepadnaviridae: Hepatitis B virus (HBV) 2.6. Baculoviridae: Nuclear polyhedrosis virus (NPV) and Granulosis virus (GV) 3. NUCLEAR ENVELOPE TARGETING 3.1. Herpes simplex virus type 1 (HSV-1) 3.2. Nuclear polyhedrosis virus (NPV) 3.3. Other viruses 4. NUCLEAR IMPORT: UNCOATING, DOCKING TRANSLOCATION 4.1. Adenovirus type 2 (Ad-2) 4.2. Simian virus 40 (SV40) 4.3. Herpes simplex virus type 1 (HSV-1) 4.4. Hepatitis B virus (HBV) 4.5. Nuclear polyhedrosis virus (NPV) and Granulosis virus (GV) 5. CONCLUSIONS 6. TABLES 7. REFERENCES Abbreviations: AAV: Adeno-associated virus AcNPV: Autographa californic Nuclear polyhedrosis virus Ad: Adenovirus GV: Granulosis virus dHBV: Duck Hepatitis B virus hHBV: Human Hepatitis B virus HSV: Herpes simplex virus NPV: Nuclear polyhedrosis virus MMV: Mouse minute virus NLS: Nuclear localization sequence NPC: Nuclear pore complex NE: Nuclear envelope SV40: Simian virus 40 c: circular, ds: double stranded, kb: kilo bases, l: linear, pds: partly double-stranded, ss: single stranded 2 1. INTRODUCTION Viruses are natural carriers of genetic information between cells. They are made up of proteins, nucleic acids and often lipids. Viruses are usually smaller than ribosomes, but can be as big as a bacterial cell. Their DNA or RNA genome is tightly packed and well protected with proteins and often enwrapped with a lipid envelope. Within a virus particle, which is outside a host organism, the genome is inactive. The genome only becomes activated when the virus interacts with a host cell in a very specific manner. By utilizing the host cell’s mechanisms, a virus particle enters, unpacks the genome and initiates its replication and transcription programs. It finally directs the cell to assemble and release progeny virions into the extracellular milieu for a new round of infection. Viruses that infect eukaryotic cells replicate either in the cytoplasm or in the nucleus. Viruses replicating in the nucleus usually contain a DNA or, seldomly, an RNA genome. These viruses take advantage of the nuclear compartment for their DNA synthesis, for generation and processing of their mRNA and in most cases, they also assemble progeny virions within the nucleus (for review, see Fields, et al., 1996). RNA viruses replicating in the nucleus, such as the orthomyxovirus influenza virus, or the RNA lentiviruses, examplified by the human immunodeficiency virus (HIV), follow a similar strategy as the DNA viruses, but form their progeny outside the nucleus (for review, see Freed, et al., 1995, Stevenson, 1996, Trono, 1995, Whittaker, et al., 1996). For HIV, the incoming viral RNA is reverse transcribed in the cytoplasm to form linear double stranded DNA, the proviral DNA, which is then imported into the nucleus as a high molecular weight nucleo-protein complex. The DNA viruses, which do not replicate in the nucleus, such as poxviruses and iridoviruses, do not need nuclear targeting information. They must, however, contain additional genetic information to build up their own DNA and mRNA synthesis factories within the cytoplasm (for review, see Moss, 1996). The genome of DNA viruses replicating in the nucleus must cross three barriers, the plasma membrane, the cytosol and the nuclear envelope. A virus particle gets across the plasma membrane by attachment with high affinity to a suitable cell surface receptor. The cytosol is traversed either by passive diffusion in the case of small viruses or by exploitation of the cell’s motile functions in the case of large viruses. To overcome the nuclear envelope barrier nuclear DNA or RNA viruses have developed two principal strategies. The onco- retroviruses wait for the cell to break down its nuclear envelope during cell division. They then integrate their reverse transcribed DNA genome into the host chromatin (for review, see Coffin, 1996). The nuclear DNA- containing viruses, typified by the adenoviridae, parvoviridae, papovaviridae, baculoviridae, hepadnaviridae and herpesviridae have chosen to import their genome into the nucleus across an intact nuclear envelope (see Table 1). Emerging evidence suggests that DNA viruses are using elements of the same pathways, which control transport of cellular proteins and nucleic acids into the nucleus. In this review, I will survey evidence on different mechanisms of DNA virus entry. I will briefly describe the viruses and their portals of entry into cells, and then discuss various nuclear envelope targeting and DNA import mechanisms. 3 2. CELL SURFACE BINDING AND UPTAKE The first step in virus infection of a host cell is attachment to the cell surface. Attachment can be mediated by a large variety of cell surface molecules (for review of different virus receptors, see Wimmer, 1994, see also Table 2). Attachment is necessary but not always sufficient for a successful infection, since subsequent steps, such as membrane penetration and disassembly often require virus binding to specialized surface receptors. The presence of specific receptors is often an important determinant of host range and cell and tissue tropism of a virus, but other factors acting in different phases of the replication cycle can be important for productive infection as well. A variety of experimental strategies has been employed over the years to identify specific viral receptors. Most of these studies, however, have not conclusively demonstrated that a putative receptor actually serves to initiate productive virus entry. The most stringent criteria of biological significance of a putative receptor are the transformation of receptor-negative nonpermissive cells to a permissive phenotype by means of recombinant DNA technology and the concomitant block of permissiveness of the engineered cells with a specific antibody applied to the cell surface. This technology is readily applicable if the receptor is encoded by a single gene. If the receptor is a carbohydrate, one can restore the permissiveness by adding exogenous glycolipids of exactly defined composition to nonpermissive cells. In such reconstitution experiments, glycolipids have an advantage over glycoproteins, since they can contain a single carbohydrate chain and can insert themselves into the cell membranes. Emerging evidence indicates that virus binding to the cell surface is a multistep process for many viruses. Multiple receptors can either act together or sequentially. Binding to a first receptor can cause changes in the virus or the host, which then favor interactions with a second receptor. For blood- or respiratory secretions- born viruses, the initial binding must effect rapid virus docking. In this case, the rate of binding can be more important than the affinity, which is an equilibrium measurement (for review, see Williams, 1991). If the first receptor allows fast binding, the second receptor could be responsible for any slower processes, such as stable binding, endocytosis or penetration. To understand dynamic interactions between viruses and cells we need to understand questions like, how many molecules are engaged in the interactions and how long do they need to be associated to confer a biological effect. For virus-cell interactions we are just at the beginning of this new phase of understanding. NON-ENVELOPED VIRUSES 2.1. Adenovirus 2 and 5 (Ad-2 and Ad-5) Ad-2 and Ad-5 belong to the subgroup C adenoviruses. These viruses are non-oncogenic and widely used as gene transfer vehicles into somatic cells, when rendered replication defective (for review, see Yeh and Perricaudet, 1997). Adenoviruses naturally enter human airway cells and produce progeny virions within the nucleus of the infected cell (for review, see Horwitz, 1990). The Ad-2 particle is icosahedral and has a diameter of approximately 90 nm. It contains at least 11 different structural polypeptides and a linear double stranded DNA molecule, which is condensed with proteins V, VII, µ. The DNA also contains about 10 copies of the cysteine protease p23 and is covalently associated with a terminal protein at its 5’ ends (Mangel, 4 et al., 1993, Stewart, et al., 1993). The chromosome is connected to the inside wall of the capsid via protein VI. The capsid consists of 6 to 7 different proteins. More than 75% of its mass is contributed by the hexon protein. This major protein is held together by homophilic interactions and by the minor protein IX. Protein IIIa is another capsid stabilizing component linking adjacent facets of the icosahedron. The vertices of the capsid are made up of pentameric penton base and protruding trimeric fibers. The fibers are built by a linear shaft and a carboxy-terminal globular head domain of 200 amino acids containing the receptor binding determinant (Louis, et al., 1994, Xia, et al., 1995). For entry into cells, Ad-2 and Ad-5 use an immunoglobulin gene family protein, termed CAR (Coxsackie B virus, an unrelated RNA virus, and Adenovirus Receptor), as an initial attachment site (Bergelson, et al., 1997, Tomko, et al., 1997). This receptor binds with high affinity to the fiber head domain. The precise nature of the interaction, i.e., the on and off rates or amino acid residues making up the interface, are not yet known. The Ig domain is most likely directly involved in fiber binding, since recently, the Ig domain containing MHC class I protein has also been identified as a functional primary receptor for Ad-5 (Hong, et al., 1997). Besides the fiber receptor, the alpha m-beta 2 integrin of hematopoietic cells has also been proposed to function as a high affinity attachment site in the absence of fiber proteins (Huang, et al., 1996).
Details
-
File Typepdf
-
Upload Time-
-
Content LanguagesEnglish
-
Upload UserAnonymous/Not logged-in
-
File Pages30 Page
-
File Size-