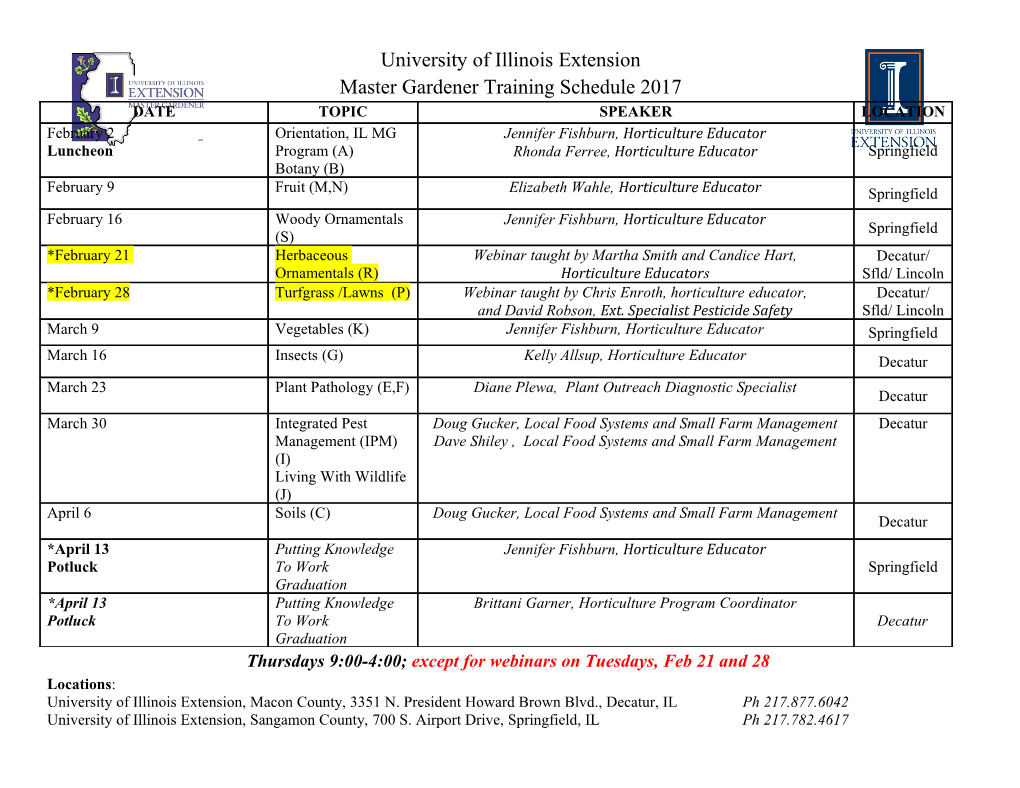
PostCFT.tex 9:09 p.m. October 5, 2013 Remarks on applications of class field theory Bill Casselman University of British Columbia [email protected] Class field theory is ubiquitous in the theory of automorphics forms. I thought it might be a good idea to show several different aspects of how it occurs. The principal theorem about Galois descent is, roughly speaking, that if E/F is a Galois extrension and A an algebraic structure defined over E, then structures over F that become isomorphic to A over E are classified by splittings of a short exact sequence 1 −→ AutE(A) −→ E −→ Gal(E/F) −→ 1 modulo conjugation by elements of AutE(A). Such splittings can be classified by certain cohomology groups 1 H (Gal(E/F ), AutE(A)). Class field theory is invariably required inevaluating such cohomology groups. Contents 1. Galois descent 2. Central simple algebras 3. Weil groups 4. Artin L•functions 5. Langlands’ classification for tori 1. Galois descent Galois descent is concerned with the question, supposing that I know what some structure is like over a separable closure of a field F , what can I say about its structure over F ? REFERENCES 1. Bill Casselman, ‘Algebraic structures and fields of definition’, available at http://www.math.ubc.ca/ cass/research/pdf/Descent.pdf 2. James Milne, Algebraic geometry, available at http://www.jmilne.org/math/CourseNotes/ag.html Chapter 16 of this book is about descent, mostly Galois but with a brief mention of Grothendieck’s notion of faithfully flat descent. This includes a theory, due to Pierre Cartier, of descent with respect to finite inseparable extensions. 3. Jean•Pierre Serre, Local fields, Springer, 1979. 4. ——, Algebraic groups and class fields, Springer, 1988. This is the standard reference for a translation of Weil’s theory into modern terms. 2. Central simple algebras Central simple algebras offer the simplest examples of phenomena of descent. The theory also describes the structure of the unit groups of central simple algebras. Central simple algebras are directly related to class field theory because equivalence classes of such algebras is in bijection with the fundamental cohomology group 2 × H (Gal(Fs/F ), Fs ). REFERENCES 5. Max Deuring, Algebren, AMS reprint. Applications of CFT (9:09 p.m. October 5, 2013) 2 This is a very readable book, particularly the last few chapters. But it is in German. 6. Andre´ Weil, Basic number theory (second edition), Springer, 1995. This might be considered the replacement for Deuring’s book, but is much more verbose. 3. Weil groups I shall look only at local Weil groups. P-ADIC. Suppose k to be a p•adic field, ks a separable closure. The Galois group Gal(ks/k) projects onto the Galois group of knr/k, which is isomorphic to the Galois group of the residue field extension F/Fq. One can m m define Wk to be the inverse image of powers of the Frobenius F (q = p ). It is dense in Gal(ks/k). There are several advantages to replacing the Galoi group by the Weil group. The principal one is that quotient of Wk by its commutator subgroup, maps into a dense subgroup of the Galois group of the maximal abelian extension of k. The reciprocity theorem for local fields asserts that there is a canonical isomorphism of this × quotient with k . Actually, there are two conventions about this reciprocity map—one has the generator ̟ of p − map to Fm, while the other has it map to the inverse F m. The structure of the Weil group contains within it much information about local class field theory. If E/F is a finite extension, the maximal abelian extension Eab/F is a Galois extension of F . We can define a relative Weil group WE/F as a dense subgroup of Gal(Eab/F ) that fits, by reciprocity, into the short exact sequence × 1 −→ E −→ WE/F −→ Gal(E/F ) −→ 1 . One of the basic results of local class field theory is that this extension corresponds to the fundamental class of H2(Gal(E/F ),Ex). In other words, class field theory has something to say about non•abelian extensions of k as well as abelian ones. This result is found originally to [Shafarevitch:1946]. Both [Artin•Tate:1968] and [Tate:1979] explain how the assembly of all Weil groups of p•adic fields encapsulates all of local class field theory for such fields. × × × REAL AND COMPLEX. The Weil group WC is just C . The Weil group WR is the normalizer of C in H , and is generated by Cx and an element σ such that σz = zσ and σ2 = −1. It fits into a short exact sequence × 1 −→ C −→ WR −→ 1 . The group WR is therefore the same as WC/R. It does not have any obvious Galois group interpretation, but it does play a Galois•theoretic role concerning certain fields associated to abelian varieties with complex multiplication. REFERENCES 7. Emil Artin and John Tate, Class field theory, Benjamin, 1968. 8. Armand Borel and Bill Casselman (editors), Automorphic representations and L•functions, Proceedings of Symposia in Pure Mathematics XXXIII, American Mathematical Society, 1979. 9. I. R. Shafarevitch, ‘On Galois groups of p•adic fields’, Doklady Akademia Nauk 53 (1946), 15–16. There is an English version in his Collected works. Shafarevitch’ paper is the first mention of what later came to be known as the Weil group, but the relationship is not transparent. He constructs it as the normalizer of a torus in a certain p•adic division algebra. This is a very basic result, but I have trouble understanding exactly how it fits in with the general theory. An appendix in the second edition of [Weil:1995] is about this result, but seems to wander around without stating the point clearly. Theorem 6 in [Artin•Tate:1968] is a version of this theorem, but as far as I can see it is not obviously equivalent. 10. John Tate, ‘Number theoretic background’, pp. 3–26 in [Borel•Casselman:1979]. A standard reference for Weil groups, although somewhat dry. Applications of CFT (9:09 p.m. October 5, 2013) 3 4. Artin L•functions Suppose E/F to be a Galois extension of algebraic number fields, unramified outside the finite set S of primes. Suppose ρ to be a representation of Gal(E/F ). If p is a prime of F , suppose P to be a prime of E over it. The IP iP representation ρ induces a representation ρ of the quotient DP/IP on V . But this quotient is isomorphic to the Galois group of residue fields, generated by what I’ll call FP. The expression 1 I −s det 1 − ρ P (FP)Np is independent of choices, because all are effectively conjugate. I’ll write it as 1 L (s,ρ)= . p I −s det 1 − ρ p (Fp)Np The product 1 L(s,ρ)= Lp(s,ρ)= − . det 1 − ρIp (F )Np s Yp Yp p converges for RE(s) > 1. ∗ If H is a normal subgroup of G and ρ is a representation of G/H, let ρ be the lifting to G. If H is any subgroup of G and ρ a representation of H, let ρ∗ be the induced representation of G. [three-artins] 4.1. Proposition. We have (a) Lp(s,ρ + σ)=Lp(s,ρ)Lp(s, σ). ∗ (b) Lp(s,ρ )=Lp(s,ρ). (c) Lp(s,ρ∗)=Lp(s,ρ). In addition, (d) if ρ is a character then Lp(s,ρ) the same as the L•function defined classically according to the reciprocity theorem. These four properties will be features of other local and global factors of L•functions. Only the last is not straightforward. I follow §3 of [Deligne:1972]. It reduces to a statement about extensions of the finite residue fields. We may assume G to be the cyclic group of order m generated by F, H to be generated by some Fn with n|m. We may first decompose ρ into irreducible one•dimensional components, and then by the sum rule assume ρ itself to be a character. The denominator of the L•function for H is thus now − 1 − ρ(Fn)Np ns = 1 − Xn − with X = ρ(F)Np s. What about L(s,ρ∗)? We can describe ρ∗(F) explicitly. I recall that ρ∗(g) is the right regular action of G on the space of all functions {f: G −→ C | f(hg)= ρ(h)f)g) for all h ∈ H,g ∈ G} . i We may choose the F with 0 ≤ i<n as representatives of G/H. These determine a basis Fi of ρ∗: ρ(h) if i = j F (hFj )= i 0 otherwise. Each coset HFi supports a one•dimension Then the action of F in ρ∗ is Fi+1 if i<n − 1 ρ∗(F): Fi 7−→ n ρ(F )F0 otherwise. The denomiantor of L(s,ρ∗) is −s det(I − ρ∗(F)Np ) . Applications of CFT (9:09 p.m. October 5, 2013) 4 − It is the characteristic polynomial det(I − MY ) with M = ρ∗(F) evaluated at Y = Np s. It follows from a well known result in linear algebra that this is the same as det(I − ρ(FnY n). If M is any linear transformation of dimension n, its characteristic polynomial is 2 2 det(I − Mt) = 1 − (TR M) t + (TR M) t + ···± det(M) tn . V Suppose n n−1 n−2 P (x)= x + an−1x + an−2x −··· + a0 to be a polynomial of degree n.
Details
-
File Typepdf
-
Upload Time-
-
Content LanguagesEnglish
-
Upload UserAnonymous/Not logged-in
-
File Pages6 Page
-
File Size-