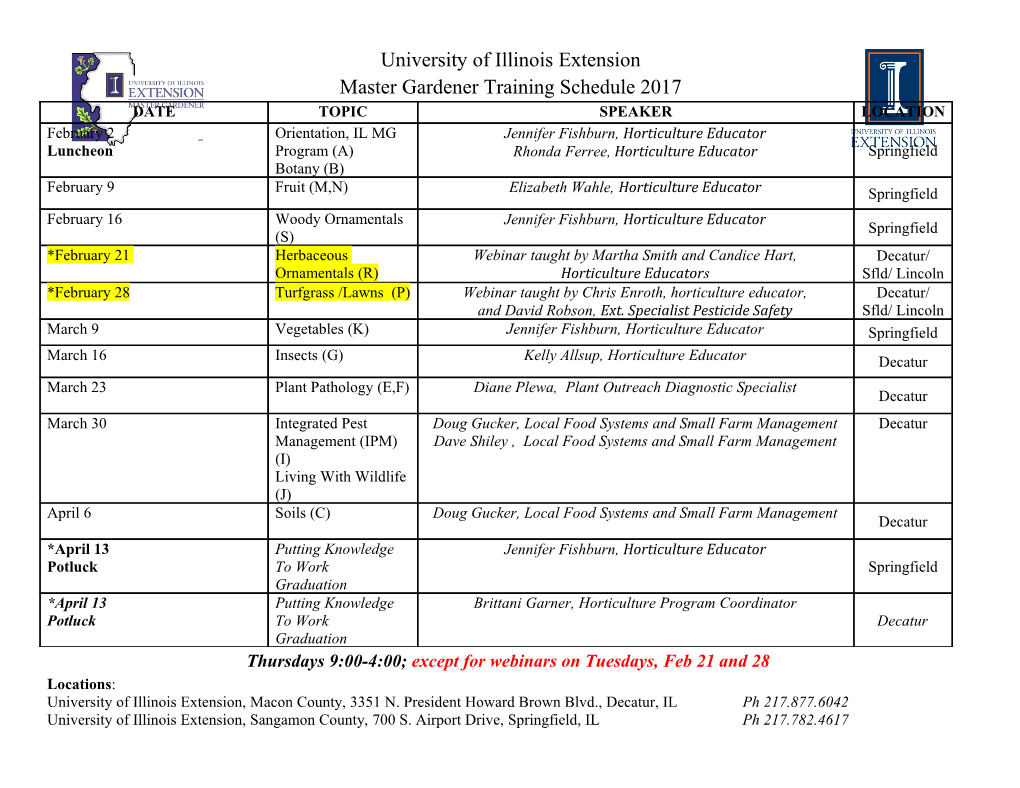
ARTICLE DOI: 10.1038/s41467-018-04780-x OPEN Twinning in metastable high-entropy alloys Shuo Huang1, He Huang1,2, Wei Li1,3, Dongyoo Kim1,4, Song Lu1, Xiaoqing Li1, Erik Holmström5, Se Kyun Kwon6 & Levente Vitos 1,3,7 Twinning is a fundamental mechanism behind the simultaneous increase of strength and ductility in medium- and high-entropy alloys, but its operation is not yet well understood, which limits their exploitation. Since many high-entropy alloys showing outstanding mechanical properties are actually thermodynamically unstable at ambient and cryogenic 1234567890():,; conditions, the observed twinning challenges the existing phenomenological and theoretical plasticity models. Here, we adopt a transparent approach based on effective energy barriers in combination with first-principle calculations to shed light on the origin of twinning in high- entropy alloys. We demonstrate that twinning can be the primary deformation mode in metastable face-centered cubic alloys with a fraction that surpasses the previously estab- lished upper limit. The present advance in plasticity of metals opens opportunities for tai- loring the mechanical response in engineering materials by optimizing metastable twinning in high-entropy alloys. 1 Applied Materials Physics, Department of Materials Science and Engineering, Royal Institute of Technology, Stockholm SE-100 44, Sweden. 2 Science and Technology on Surface Physics and Chemistry Laboratory, Mianyang 621900, PR China. 3 Department of Physics and Astronomy, Division of Materials Theory, Uppsala University, SE-75120 Uppsala, Sweden. 4 Department of Physics, Pukyung National University, Busan 608-737, Republic of Korea. 5 Sandvik Coromant R&D, 126 80 Stockholm, Sweden. 6 Graduate Institute of Ferrous Technology, Pohang University of Science and Technology, Pohang 37673, Republic of Korea. 7 Institute for Solid State Physics and Optics, Wigner Research Centre for Physics, H-1525 Budapest, Hungary. Correspondence and requests for materials should be addressed to S.H. (email: [email protected]) or to S.K.K. (email: [email protected]) or to L.V. (email: [email protected]) NATURE COMMUNICATIONS | (2018) 9:2381 | DOI: 10.1038/s41467-018-04780-x | www.nature.com/naturecommunications 1 ARTICLE NATURE COMMUNICATIONS | DOI: 10.1038/s41467-018-04780-x esigning strong, and at the same time, ductile metals has Here, we employ first-principle quantum mechanical tools to been among the most ambitious goals for metallurgists. resolve the twinning mechanism in metastable multi-component D fl ≤ ≤ The apparently con icting mechanisms often resulted in alloys. We select the representative Fe80−xMnxCo10Cr10 (30 x grades compromising between strength and ductility. In spite of 45) and CrMnFeCoNi HEAs3,6, and CrCoNi MEA7, for which the early ideas revolving around Fe–Mn alloys1, simultaneous reliable experimental data for the deformation mechanisms exist. improvement of the two properties has been very challenging. Based on the intrinsic energy barriers, we show that it is the The discovery of the advanced high-strength steels near the turn particular chemistry that is primarily responsible for the emer- of the century was a pioneering step towards reaching such gence of twinning in metastable alloys, and decreasing tempera- goals2. Recently, the strength–ductility tradeoff was also over- ture efficiently drives the system across various deformation come by designing dual-phase high-entropy alloys (HEAs)3, modes. The inherent affine shear strain prior to slip, which is which turned attention towards non-conventional alloys con- associated to the relatively large critical twinning stress char- taining multi-principal elements in equal or nearly equal con- acteristic to the present systems, further promotes twinning. Our centrations4,5. Successful single-phase solutions also exist such as results are expected to help optimizing the properties of engi- the fracture-resistant HEAs6 and the exceptional damage- neering materials involving HEAs towards excellent tolerance medium-entropy alloys (MEAs)7. Further, HEAs with strength–ductility combinations. surprising and unanticipated properties are being continuously – put forward8 18. Parallel with the experimental efforts, compu- tational modeling was also advanced to optimize compositions Results and reveal the atomic-level mechanisms19 behind the strength Deformation modes. The leading deformation modes in and ductility improvements. fcc metals are stacking fault (SF), twinning (TW), and full- The common denominator of the most promising routes over slip (SL). The active mode is decided by the competition the strength–ductility dilemma is the tailored plastic deformation between the corresponding effective energy barriers (EEBs): γ θ γ = θ γ θ γ γ = θ γ θ mechanism. Face-centered cubic (fcc) metals normally deform by SFðÞ¼ usf cos , TWðÞ¼ðutf À isf Þ cos , and SLðÞ¼ γ γ = θ 27 dislocation glide resulting in good ductility and reduced strength. ð usf À isf Þ cosð60 À Þ for SF, TW, and SL, respectively .In But in the aforementioned special alloys, the leading deformation these expressions, θ varies between 0° and 60° and reflects the modes are twinning and transformation induced plasticity. In effect of grain orientation relative to the resolved shear direction, γ γ γ particular, twinning was found to play an important role for the isf is the intrinsic SFE, usf the unstable SFE, and utf the enhanced ductility and high strain hardening rate2,3,6,7,20,21. unstable twin fault energy. These intrinsic material parameters There are several phenomenological plasticity models22,23 devel- (IMPs) describe the generalized SFE (γ-surface)39 and are oped to understand the activation of twinning deformation in fcc determined from a series of ab initio calculations. According to γ θ γ θ metals. These models are based on the stacking fault energy (SFE) the above EEBs, twinning is possible for TWðÞ SFðÞ, defined as the excess energy due to the stacking fault connecting otherwise martensitic transformation occurs. Furthermore, due to the Shockley partial dislocations. For instance, austenitic steels the shear direction dependence, SF and SL or TW and SL can co- − with SFE higher than 10–16 mJ m 2 are predicted to show exist in a homogenous material, but SF and TW are exclusive to – twinning24 26, whereas alloys with SFE below this critical value each other independently of θ (they possess similar Schmid fac- should exhibit deformation-induced phase transformation. The- tors). We mention that SF and TW can coexist in special alloys – – oretical works also addressed the question of twinnability27 29, (e.g., interstitial HEAs16 18) due to the broadening of their and they indicate twinning for fcc stable metals with twinning boundary. For the sake of simplicity, in the following we do not fraction below 50%27. always mention SL when discussing the SF and TW mechanisms. Within the simplest model, the SFE corresponds to the energy Using the calculated EEBs, first we explore the deformation difference between the hexagonal close-packed (hcp) and fcc modes of the Fe80−xMnxCo10Cr10 alloys as a function of structures30. Thus, the sizable positive SFE required for twinning temperature and composition. These alloys are reported to by the phenomenological models22,23 implies a thermo- exhibit twinning or martensitic transformationÀÁ depending on Mn 3 γ γ γ dynamically stable fcc phase. Traditional alloys like austenitic content . The room-temperature SF À TW SFðÞÀ0 31 fi γ steels belong to this group. Since the discovery of the rst fcc TWðÞ0 valuesÀÁ are plotted in Fig. 1 as a function of Mn content. 4,5 fi γ γ HEAs based on 3d transition metals , it was expected that these We nd that SF À TW changes sign with increasing Mn multi-component systems also follow a similar plastic deforma- concentration. Namely, for x ≥ 33, TW is favored against SF, tion scenario. However, recent experimental works reported a whereas for x < 33 SF is activated. In addition, SL is present in pressure-induced fcc–hcp transition in CrMnFeCoNi, and the both regimes with θ increasing towards 60° (not shown). These hcp phase was retained after decompression to ambient pres- theoretical predictions are in perfect agreement with observa- sure32,33. The observed equilibrium phase boundary between the tions3 and confirm that the present theory correctly captures the fcc and hcp phases was placed near 600 K33. For the multi- observed trends of the deformation modes as a function of – component alloys in question3,6,7,20,34 37, including CrMnFe- composition. fi fi Δ CoNi, ab initio theory fully con rms the experimental ndings Figure 1 also shows the free energy difference F ðFhcp À Δ by predicting a thermodynamically unstable fcc lattice at low FfccÞ for Fe80−xMnxCo10Cr10 by varying x. Negative F indicates a temperature. Hence, both experiment and theory suggest that thermodynamically unstable fcc lattice, which turns out to be the the metastable fcc CrMnFeCoNi alloy should possess very small case for all compositions consideredÀÁ here. By combining the Δ γ γ positive or negative SFEs at ambient and cryogenic conditions. behavior of F with that of SF À TW in terms of Mn content, But such SFE values in connection with the phenomenological we arrive to a very interesting and unprecedented phenomenon. 22,23 6 models clearly fail to explain the observed twinning . Namely, in Fe80−xMnxCo10Cr10 HEAs, the room-temperature Thus, the available experimental and theoretical evidences twinning is associated with a metastable fcc phase for 33 ≤ x ≤ 50 severely
Details
-
File Typepdf
-
Upload Time-
-
Content LanguagesEnglish
-
Upload UserAnonymous/Not logged-in
-
File Pages7 Page
-
File Size-