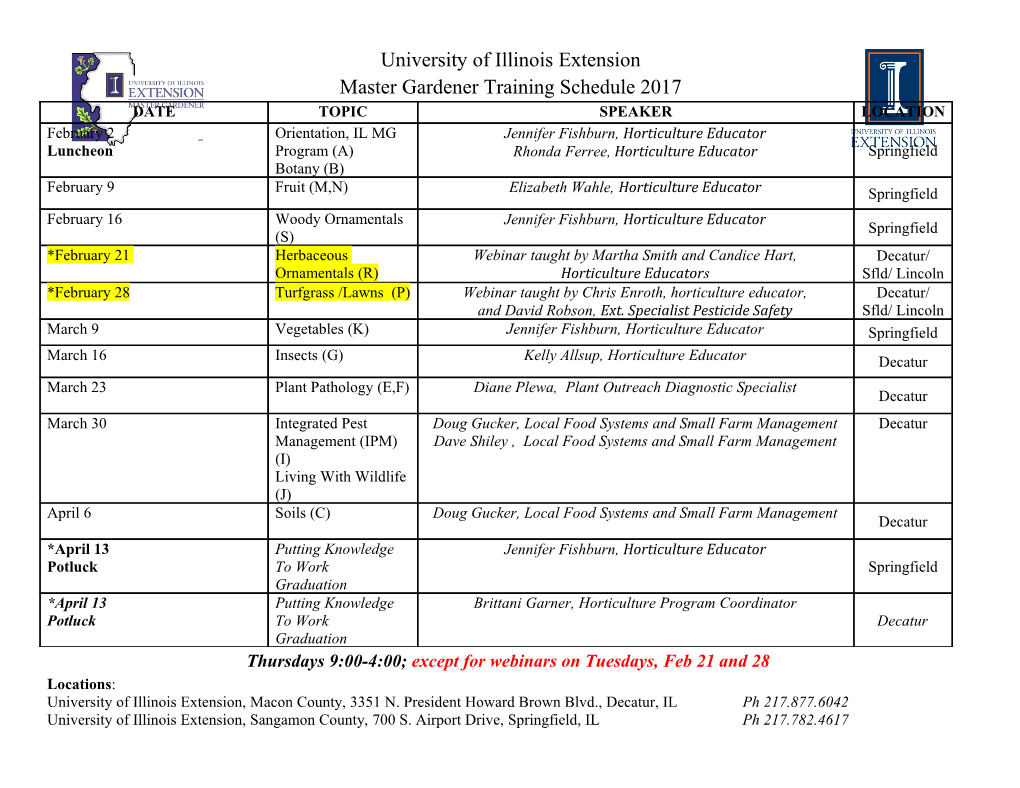
FAKULTÄT FÜR MATHEMATIK UND NATURWISSENSCHAFTEN FACHGRUPPE PHYSIK BERGISCHE UNIVERSITÄT WUPPERTAL Scintillator Calorimeters for a Future Linear Collider Experiment Dissertation by Oskar Hartbrich July 22, 2016 Contents Introduction 7 1. Particle Physics9 1.1. The Standard Model................................9 1.2. Future e+e- Experiments.............................. 11 1.2.1. The International Linear Collider..................... 13 1.2.2. The International Large Detector..................... 15 1.2.3. Model Independent Determination of Higgs Parameters at the ILC. 17 1.3. Particle Interactions with Matter......................... 25 1.3.1. Electromagnetic Cascades......................... 25 1.3.2. Heavy Charged Particles......................... 27 1.3.3. Hadronic Cascades............................. 28 1.4. Simulation of Particle Showers.......................... 31 1.4.1. Electromagnetic Cascades......................... 31 1.4.2. Hadron Cascade Simulations....................... 31 1.4.3. Physics Lists................................. 33 1.5. Calorimeters..................................... 34 1.5.1. Particle Flow Calorimetry......................... 38 2. CALICE Physics Prototypes 41 2.1. Silicon Photomultipliers.............................. 41 2.2. The Scintillator Electromagnetic Calorimeter.................. 44 2.3. The Analog Hadron Calorimeter......................... 44 2.4. The Tail Catcher and Muon Tracker........................ 45 2.5. DAQ and Electronics of the Scintillator-SiPM Prototypes........... 46 2.6. Calibration of the CALICE Scintillator-SiPM Prototypes............ 47 2.6.1. Pedestal................................... 47 2.6.2. SiPM & Tile Properties........................... 48 2.6.3. MIP Calibration............................... 49 2.7. Digitisation Effects................................. 50 2.7.1. Hit Timing.................................. 50 2.7.2. Optical Crosstalk.............................. 50 2.7.3. Conversion to the MIP Scale....................... 51 2.7.4. Scintillator-SiPM Modelling........................ 51 2.7.5. Noise..................................... 56 3. CALICE Engineering Prototypes 57 3.1. The AHCAL Engineering Prototype....................... 57 3.1.1. The HCAL Base Unit............................ 58 3 4 Contents 3.1.2. The SPIROC2 ASIC............................. 58 3.1.3. Current Status of the AHCAL Engineering Prototype......... 60 3.2. Considerations for a Full Scale Calorimeter System............... 61 3.2.1. Tile Lightyield................................ 62 3.2.2. Trigger Threshold Validation....................... 64 3.2.3. SPIROC2 Readout Efficiency....................... 67 4. Energy Resolution of the Combined Scintillator Calorimeter System 71 4.1. The FTBF MTest Beamline............................. 71 4.2. Testbeam Setup................................... 72 4.3. Simulation Setup.................................. 75 4.3.1. ScECAL Absorber Composition..................... 75 4.3.2. Beam Profiles................................ 76 4.3.3. Relative Alignment of the Subdetectors................. 81 4.4. Reconstruction.................................... 82 4.4.1. Deposited Energy.............................. 82 4.4.2. Layer of First Interaction.......................... 83 4.4.3. Track Reconstruction............................ 88 4.5. Event Selection................................... 90 4.5.1. MIP Selection................................ 92 4.5.2. Pion Selection................................ 92 4.5.3. Electron Selection.............................. 97 4.5.4. Pion Event Contaminations........................ 103 4.6. Validation Studies.................................. 107 4.6.1. MIP Calibration............................... 108 4.6.2. Hit Energy Spectra and Shower Profiles................. 110 4.6.3. ScECAL Electron Response & Resolution................ 111 4.7. Energy Reconstruction in a Combined Calorimeter System.......... 114 4.7.1. Standard Weighting............................ 116 4.7.2. Software Compensation Weighting.................... 116 4.8. Pion Analysis.................................... 129 4.8.1. Pion Shower Profiles............................ 129 4.8.2. Energy reconstruction and linearity................... 131 4.8.3. Energy resolution.............................. 132 4.8.4. Applying the Software Compensation Weights from Simulation to Data133 4.9. Summary and Outlook............................... 136 5. Validation of the HCAL Simulation for ILD 139 5.1. Simulation Setup.................................. 139 5.1.1. Model Geometry Modifications...................... 139 5.1.2. ILDCaloDigi................................. 140 5.2. Digitisation Effects on Shower Measurement.................. 141 5.3. Testbeam Comparison............................... 145 5.4. Conclusions..................................... 147 Summary 149 Contents 5 Acknowledgements 153 Appendix 155 A. Analytical Resolution of the Binomial SiPM-Model............... 155 B. Systematic Influence of the Observed Beam Contamination in Pion Runs.. 156 C. Event Selections................................... 158 D. Additional Information FNAL Testbeam Analysis............... 161 Bibliography 173 Introduction Modern particle physics experiments measure the results of high energy particle collisions. The highest energy particle accelerator to date is the Largep Hadron Collider (LHC) at CERN, colliding protons with center of mass energies up to s = 14 TeV. The discovery of the Higgs boson at two independent experiments at the LHC in 2012 completed the Standard Model of particle physics and marks a cornerstone in the research of fundamental particles and their interactions. A high energy lepton collider would complement the results expected from LHC, both in achievable precision and projected discovery reach. One concept for such a facility is the International Linear Colliderp (ILC), colliding electrons and positrons with a planned center of mass energy up to s = 500 GeV. To achieve the best possible measurement precision in experiments at the ILC, unprecedented resolutions of the detector systems are required. Detector concepts planned for the ILC aim to measure jet energies to around 3 % to 4 % precision by applying Particle Flow Algorithms (PFAs). PFAs aim to combine the measurements of the tracking system and calorimeters into an optimal jet energy measurement by individually reconstructing every particle in a given jet and using the best resolution sub-detector measurement for each particle, requiring calorimeter systems with unprecedented spatial granularities. This thesis discusses multiple aspects of the ongoing developments of such highly gran- ular calorimeters within the CALICE collaboration, focusing on prototypes of scintillator based calorimeters with Silicon Photomultiplier (SiPM) readout. The discussed topics range from the testbeam analysis of a combined prototype calorimeter system, to considerations about the operation of fully integrated calorimeter prototypes in testbeam environments and a full ILC experiment, up to the performance of the hadronic calorimeter implemented in the full detector simulation of an ILC detector concept. The first chapter gives a brief recapitulation of the Standard Model and a description of the ILC and its detector concepts. Furthermore, examples for precision measurements of properties of the Higgs boson are given. Finally an introduction to interactions of particles with matter, and calorimetry is presented, including a description of the particle flow concept and its requirements. The second chapter describes the first generation calorimeter prototypes developed within the CALICE collaboration with a focus on the scintillator-SiPM technology, including a description of the calibration procedures developed for the scintillator-SiPM prototypes. The statistical models used to realistically include sensor effects into simulations are fully validated as part of this thesis. The second generation of CALICE scintillator-SiPM calorimeters is discussed in chapter three. The goal of these prototypes is to demonstrate a hardware system that is scalable to the size and demands of a full calorimeter system at a future linear collider detector. The efficient operation of such self-triggered detectors demands careful considerations about 7 8 Contents the design parameters of the whole readout chain. Some of the design parameters and their efficient validation, as well as general considerations about the expected readout efficiency of the engineering prototype system in testbeam environments and at a future linear collider experiment are investigated in this thesis. These studies are important in the current phase of continuously growing second generation CALICE prototypes, as problems identified and investigated in the current state can be mitigated and efficient solutions devised before scaling to the next larger prototypes. The fourth chapter presents testbeam analysis results obtained as part of this thesis, using data from the combined scintillator-SiPM calorimeter system consisting of the Scintillator Electromagnetic Calorimeter (ScECAL), Analogue Hadronic Calorimeter (AHCAL) and Tail Catcher & Muon Tracker (TCMT) in the energy range 4–32 GeV, recorded during a testbeam campaign at the Fermilab testbeam facility in 2009. The sampling fraction and material composition of the ScECAL is very different from the AHCAL and TCMT, complicating the reconstruction of shower energies and potentially degrading the achievable energy resolution of hadronic
Details
-
File Typepdf
-
Upload Time-
-
Content LanguagesEnglish
-
Upload UserAnonymous/Not logged-in
-
File Pages182 Page
-
File Size-