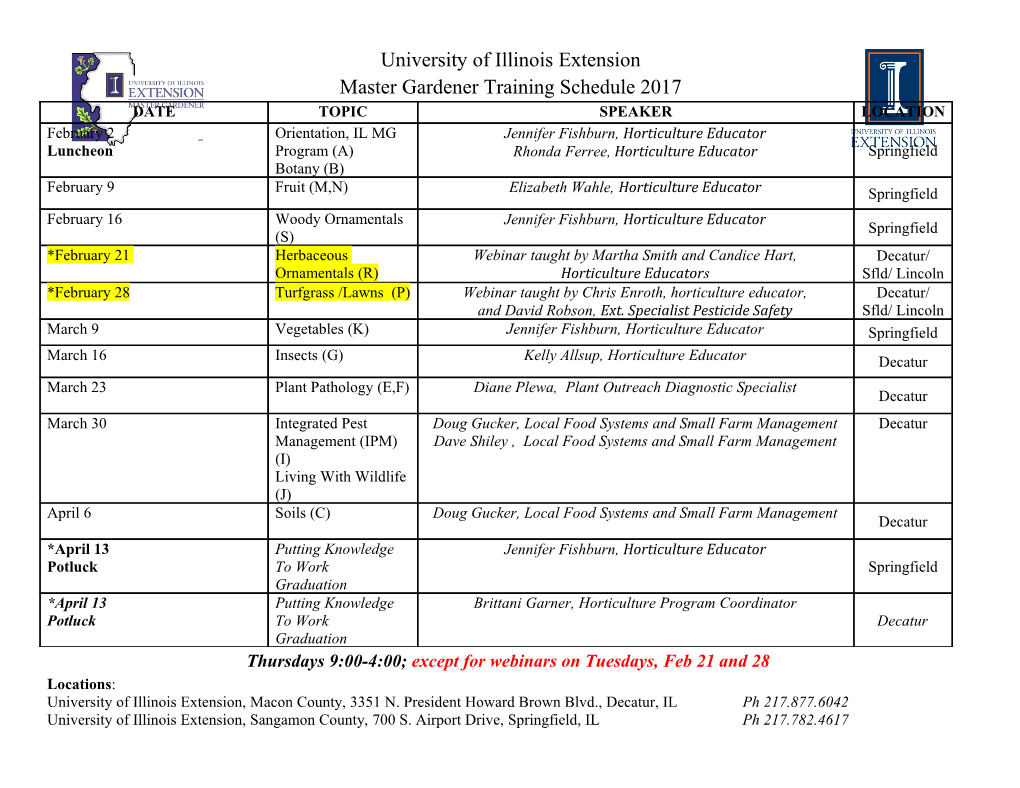
UCLA UCLA Electronic Theses and Dissertations Title Dendritic Spine Dynamics in Retrosplenial Cortex During Spatial Learning Permalink https://escholarship.org/uc/item/2742p0c5 Author Frank, Adam Publication Date 2015 Peer reviewed|Thesis/dissertation eScholarship.org Powered by the California Digital Library University of California UNIVERSITY OF CALIFORNIA Los Angeles Dendritic Spine Dynamics in Retrosplenial Cortex During Spatial Learning A dissertation submitted in partial satisfaction of the requirements for the degree Doctor of Philosophy in Neuroscience by Adam Charles Frank 2015 © Copyright by Adam Charles Frank 2015 ABSTRACT OF THE DISSERTATION Dendritic Spine Dynamics in Retrosplenial Cortex During Spatial Learning by Adam Charles Frank Doctor of Philosophy in Neuroscience University of California, Los Angeles, 2015 Professor Alcino Jose Silva, Chair Molecular and electrophysiological studies find convergent evidence suggesting that plasticity within a dendritic tree is not randomly dispersed, but clustered into functional groups. In silico neuronal modeling further suggests that clustered plasticity is able to increase storage capacity 45 times compared to dispersed plasticity. Recent in vivo work utilizing chronic two-photon microscopy tested this clustered plasticity hypothesis and showed that repetitive motor learning is able to induce clustered addition of new dendritic spines in primary motor cortex; moreover, clustered spines are more stable than non-clustered spines, suggesting a physiological role for spine clustering in learning. In this dissertation I further test the clustered plasticity hypothesis while also examining the spatial distribution of dendritic spine gain and loss. Additionally, I explore the hypothesis that spine turnover prior to learning is indicative of the plasticity within a neuronal network and thus impacts future learning and memory. To accomplish this, I utilized in vivo two-photon microscopy in Thy1-YFP-H mice to chronically image dendritic spine dynamics in retrosplenial cortex (RSC) during spatial learning. RSC is a key component of an extended spatial learning and memory circuit that includes hippocampus and entorhinal cortex. Importantly, RSC is known from lesion, immediate early gene expression, and optogenetic activation studies to be critically involved broadly in spatial learning ii and more specifically in contextual fear conditioning. I thus utilized a modified contextual fear conditioning protocol that creates gradual increases in context freezing. Behavioral training was coupled with four in vivo imaging sessions that allowed me to examine spine dynamics before training, early in learning, and after animals had reached behavioral asymptote. Results from these studies show that contextual learning induces a significant increase in the formation of clusters of new dendritic spines and that the number of new clustered spines correlates with behavioral performance; specifically, animals with the highest proportion of new spines formed in clusters also have the highest context freezing. These results support the hypothesis that spine clustering is a general mechanism for learning-related information storage in the brain and a mechanism specifically operating in the RSC. Furthermore, I find that spines lost during learning preferentially occur near clustered new spines and that spine loss also correlates with memory. Finally, I find that spine turnover before learning correlates with future learning and with clustered spine addition. From these data, I propose a model where baseline spine turnover reflects the potential for a given animal to establish new synaptic contacts during learning and clustering represents a mechanism to stabilize these connections. iii The dissertation of Adam Charles Frank is approved. Dean Buonomano Michael Fanselow Kelsey Martin Thomas O’Dell Joshua Trachtenberg Alcino Jose Silva, Committee Chair University of California, Los Angeles 2015 iv Table of Contents ABSTRACT OF THE DISSERTATION ....................................................................................... II LIST OF FIGURES .................................................................................................................. VII LIST OF TABLES .................................................................................................................... VIII ACKNOWLEDGEMENTS ......................................................................................................... IX VITA ........................................................................................................................... X CHAPTER 1 INTRODUCTION ................................................................................................ 1 1.1 Biochemical Studies of Clustered Plasticity ....................................................................................... 2 1.2 Electrophysiological Studies of Clustered Plasticity ........................................................................... 8 1.3 Structural Plasticity and Dendritic Spines ........................................................................................ 12 1.4 Studies of Clustered Structural Plasticity ......................................................................................... 16 1.5 Computational Studies of Clustered Plasticity ................................................................................. 21 1.6 Goals of the Dissertation .................................................................................................................. 23 1.7 References ....................................................................................................................................... 25 CHAPTER 2 SPATIAL LEARNING INDUCES CLUSTERED SPINE FORMATION IN RETROSPLENIAL CORTEX .....................................................................................................32 2.1 Introduction ....................................................................................................................................... 32 2.1.1 Retrosplenial Cortex ................................................................................................................. 32 2.1.2 Rationale ................................................................................................................................... 36 2.2 Methods ............................................................................................................................................ 37 2.3 Results ............................................................................................................................................. 42 2.4 Discussion ........................................................................................................................................ 52 2.5 References ....................................................................................................................................... 55 CHAPTER 3 LEARNING ALTERS THE SPATIAL DISTRIBUTION OF DENDRITIC SPINES ... ..........................................................................................................................61 3.1 Introduction ....................................................................................................................................... 61 v 3.2 Methods ............................................................................................................................................ 62 3.3 Results ............................................................................................................................................. 64 3.4 Discussion ........................................................................................................................................ 70 3.5 References ....................................................................................................................................... 74 CHAPTER 4 SPINE TURNOVER BEFORE TRAINING PREDICTS FUTURE LEARNING AND LEARNING-RELATED SPINE DYNAMICS ...............................................................................75 4.1 Introduction ....................................................................................................................................... 75 4.2 Methods ............................................................................................................................................ 76 4.3 Results ............................................................................................................................................. 77 4.4 Discussion ........................................................................................................................................ 78 4.5 References ....................................................................................................................................... 80 CHAPTER 5 CONCLUSIONS ................................................................................................83 5.1 Integration of Results ....................................................................................................................... 83 5.2 Future Directions .............................................................................................................................. 85 5.3 References ....................................................................................................................................... 88 vi List of Figures Figure 1.1: Hypothetical temporal
Details
-
File Typepdf
-
Upload Time-
-
Content LanguagesEnglish
-
Upload UserAnonymous/Not logged-in
-
File Pages103 Page
-
File Size-