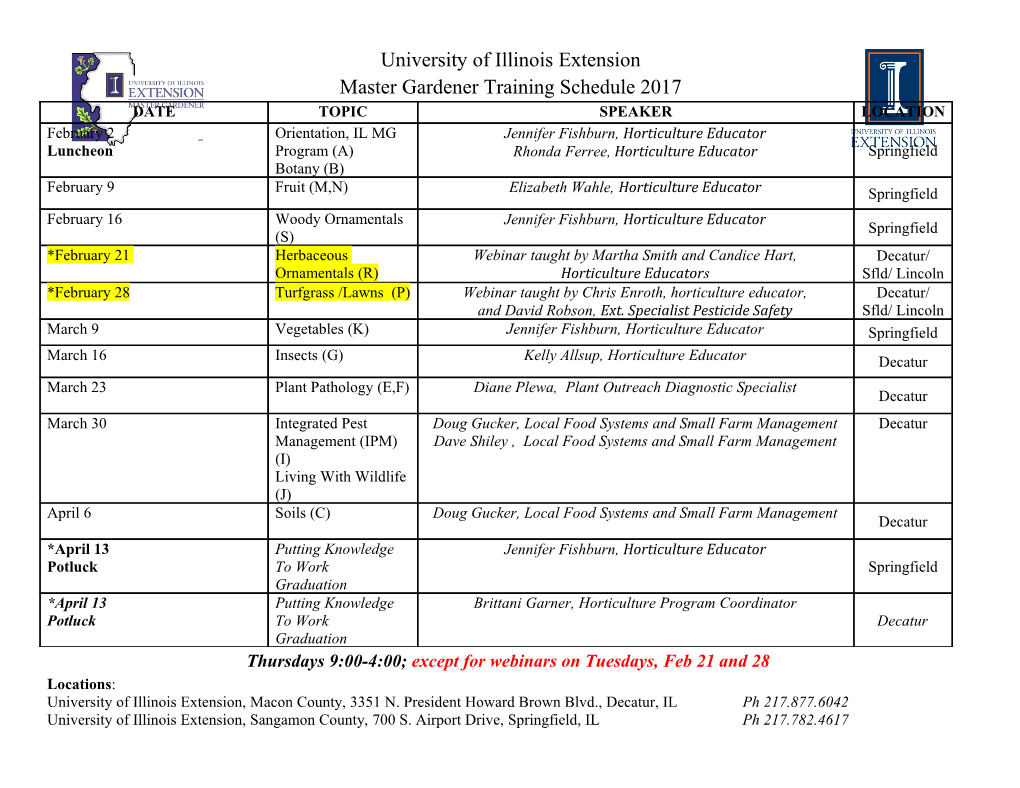
ARTICLE https://doi.org/10.1038/s41467-020-17224-2 OPEN Upgrading ketone synthesis direct from carboxylic acids and organohalides ✉ Rehanguli Ruzi1,3, Kai Liu1,3, Chengjian Zhu1,2 & Jin Xie 1 The ketone functional group has a unique reactivity in organic chemistry and is associated with a number of useful reactions. Catalytic methods for ketone synthesis are continually being developed. Here, we report a photoredox, nickel and phosphoranyl radical synergistic 1234567890():,; cross-electrophile coupling of commercially available chemicals, aromatic acids and aryl/alkyl bromides. This allows for concise synthesis of highly functionalized ketones directly, without the preparation of activated carbonyl intermediates or organometallic compounds, and thus complements the conventional Weinreb ketone synthesis. Use of the appropriate photo- catalyst, ligand amount and solvents can match the reaction rate required by any simple catalytic cycle. The practicality and synthetic robustness of the reaction are illustrated by the facile synthesis of complex ketones from readily available feedstock chemicals. 1 State Key Laboratory of Coordination Chemistry, Jiangsu Key Laboratory of Advanced Organic Materials, Chemistry and Biomedicine Innovation Center (ChemBIC), School of Chemistry and Chemical Engineering, Nanjing University, Nanjing 210023, China. 2 College of Chemistry and Molecular Engineering, ✉ Zhengzhou University, Zhengzhou 450001, China. 3These authors contributed equally: Rehanguli Ruzi, Kai Liu. email: [email protected] NATURE COMMUNICATIONS | (2020) 11:3312 | https://doi.org/10.1038/s41467-020-17224-2 | www.nature.com/naturecommunications 1 ARTICLE NATURE COMMUNICATIONS | https://doi.org/10.1038/s41467-020-17224-2 etones play a prominent role in organic chemistry. The precursors is a challenge in the oxidative addition step as a ketone moiety is extremely common in natural products result of the strong bond dissociation energy of the C–O bond K 1 fl −1 33 and pharmaceuticals and in dyes, fragrancies and a- (106 kcal mol ) . vors2. It is also a versatile reaction center in organic synthesis3. A proposed mechanism for the designed metallaphotoredox Many frequently used reactions, including the Mannich reac- cross-electrophile coupling is shown in Fig. 2. The photo- * 1/2 * III II = tion, Wittig reaction, Grignard reaction, Passerini reaction, excited [Ir(dF(CF3)ppy)2(dtbbpy)]PF6 [ Ered ( Ir /Ir ) Baeyer–Villiger oxidation, and Wolff–Kishner–Huang reduc- +1.21 V vs SCE, τ = 2.3 μs]38 causes single-electron transfer tion describe a wide array of transformations of ketones. The development of a practical route to ketones from feedstock chemicals has long been a subject of interest4–12. Carboxylic acids and organohalides are commercially abundant and a Carboxylic acid Organohalide structurally diverse, bench-stable feedstock chemicals com- monly used in organic synthesis (Fig. 1a). When producing O O Br Br ketones from carboxylic acids and organohalides, the stoi- OH CN N OH Br chiometric approach requires preparation of necessary inter- H N mediates such as amides or aldehydes and Grignard reagents13,14. If aldehydes are employed, reoxidation is neces- Abundant Feedstocks Commerical Simple Abundant Diverse sary15. Catalytic strategies for production of ketones rely on transition metal-catalyzed carbon–carbon coupling between b activated carbonyls such as acid chlorides or anhydrides with Cross-electrophile coupling Ni – organometallic reagents (Fig. 1b)16 19. However, activated O hv O e– carbonyls are generally prepared in as many as three steps from Ph3P δ+ OH + Br δ+ - carboxylic acids and organometallics are obtained typically by δ This work metalation of organohalides20, which can often lead to poor functional group compatibility or a lengthy functional group 1–3 steps 1–2 steps (activation) (reduction) protection/deprotection process. In recent years, nickel-catalyzed cross-electrophile coupling O Conventional methods 21–30 (Weinreb ketone synthesis) has attracted considerable attention .Thecarbon–carbon M δ- coupling arises from two different electrophiles in the pre- δ+ X (Reoxidation if X= H) M= Mg, Sn, B, Zn senceofstoichiometricreductant.Wepositedthatacar- Activated carbonyl boxylic acid could be directly used as a latent electrophile (C- X = R2N, Cl, terminus) rather than a nucleophile (O-terminus) in cross- OR and H coupling. This could simplify and upgrade ketone synthesis c from carboxylic acids and organic halides13.Veryrecently,our C–O formation C–C formation group and Doyle et al. reported an elegant photoredox- promoted mild deoxygenation of carboxylic acids generating n Ligand Oxidative n+1 31–35 – O Ni n O Ni acyl radicals . Since photoredox and nickel-catalyzed C O Exchange Ni Addition O bond formation between carboxylic acids and aromatic bro- C k1 X k2 C mides has been reported36 to achieve the desired cross- C electrophile coupling, acyl radical oxidative addition by a (Ref. 36: Science, 2017, 355, 380) This work metallaphotoredox pathway37–41 is essential to suppress the C–O bond formation (Fig. 1c). Alternatively, a judicious Fig. 1 Catalytic cross-electrophile coupling between carboxylic acids strategy reported by Gong et al. is conversion of carboxylic and organohalides. a The abundant feedstock chemicals in synthetic lab. acid into anhydrides in situ to suppress the C–O coupling22,23. b Direct cross-electrophile coupling of acid and organohalides. c Key However, the use of free carboxylic acids as acyl radical challenge: C–O versus C–C formation. Ar-X Ph3P II O Ir Ar LNi0 RCOOH SET Ph P Ph P II 3 O R 3 LNi X O II Base I III β R -scission III Nickel Ph3PO *Ir Photoredox SET catalysis catalysis o.a. O Ar R LNiI O LNiIII IrIII hv R r.e. X O IV R Ar Fig. 2 Proposed mechanism. Mechanistic proposal for cross-electrophile coupling of acid and aryl bromides. o.a. oxidative addition, r.e. reductive elimination. 2 NATURE COMMUNICATIONS | (2020) 11:3312 | https://doi.org/10.1038/s41467-020-17224-2 | www.nature.com/naturecommunications NATURE COMMUNICATIONS | https://doi.org/10.1038/s41467-020-17224-2 ARTICLE Table 1 Optimization of the reaction conditions. Entry Variation of standard conditions Isolated yield: 3 (3′) 1 None 82% (16%) 2 10 mol% L1 46% (37%) 3 15 mol% L1 23% (53%) 4 3 mol% of L1 19% (10%) 5 5 mol% L2 31% (33%) 6 5 mol% L3 42% (28%) 7 5 mol% L4 35% (40%) 8 5 mol% L5 nd 9 5 mol% L6 nd 10 DCM instead of DMF/MeCN nd 11 DMA instead of DMF/MeCN 25% (17%) 12 no PC or NiBr2 or Ph3P or light nd Standard conditions: photocatalyst (2 mol%), NiBr2·dme (3 mol%), L1 (5 mol%), 1a (0.2 mmol), 2a (0.4 mmol), Ph3P (0.3 mmol), K3PO4 (0.2 mmol), Cs2CO3 (0.2 mmol), DMF-CH3CN (2.0 mL, v/v = 1:1), blue LEDs, ambient temperature, 20 h. DMF N,N-dimethylformamide; DMA N,N-dimethylacetamide, DCM dichloromethane, DME 1,2-dimethoxyethane, nd not detected. =+ (SET) oxidation of triphenylphosphine (Ered 0.98V vs t t 31 – CF + PF Bu Bu SCE) ,asindicatedbyourStern Volmer experimental results F 3 6 (see Supplementary Fig. 7). The triphenylphosphine radical tBu cation (I) generated recombines with the carboxylate anion to N II N N form a phosphoranyl radical intermediate ( ).Owingtothe N L1 fi F MeO OMe strong af nity between the phosphoranyl radical and oxygen, a Ir facile β-scission of the radical species (II) occurs, giving rise to F a nucleophilic acyl radical42, which can undergo oxidative N II III III N N N addition to the resulting aryl-Ni species ( ) giving the Ni tBu species (IV)43–45. Finally, reductive elimination from the NiIII Me L2 Me IV F intermediate ( ) can generate the desired cross-electrophile CF3 II coupling product, R-CO-Ar. A second SET event from Ir Photocatalyst (PC) ([IrIII/IrII] = −1.37 V vs SCE), leading to an NiI species com- N N pletes both catalytic cycles. The synergistic combination of L3 photoredox with nickel catalysis proposed in Fig. 2 is a subject F3C CF3 of continuing research46–58, and several challenges remain in O N this cross-electrophile coupling and must be addressed: (1) N Efficient control of the matching of the C–O bond cleavage and N N N N N O the radical addition to the nickel center; (2) weakening of the L4 L5 L6 interference of stoichiometric triphenylphosphine in the nickel catalytic unit; and (3) use of appropriate ligands and solvents to Fig. 3 Photocatalysts and ligands. Catalysts and ligands for cross- significantly suppress the C–O bond formation. Herein, we electrophile coupling of acid and aryl bromides. report a formal cross-electrophile coupling of carboxylic acids with aryl or alkyl halides enabled by photoredox and nickel catalysis, and phosphoranyl radical synergistic chemistry, The optimized reaction conditions include 2 mol% [Ir{dF(CF3) leading to concise synthesis of ketones (Fig. 1b). ppy}2{dtbbpy}]PF6,3mol%NiBr2.dme together with 5 mol% ′ ′ L1 4,4 -di-tert-butyl-2,2 -bipyridine ( ,Fig.3)and1.5equivPh3P Results with a mixed DMF-CH3CN solvent (entry 1, Table 1). Under Reaction optimization. Our investigation of this cross- the standard conditions, the desired cross-electrophile coupling 3 electrophile coupling began with the reaction of 4-methyl- product ( ) can be obtained in 82% yield while the yield of the – 3′ benzoic acid (1) with 5-bromo-2-(trifluoromethyl) pyridine (2), C O coupling process, giving is suppressed to 16%. We and the representative results are presented in Table 1. found the loading amount of ligand plays an important role in NATURE COMMUNICATIONS | (2020) 11:3312 | https://doi.org/10.1038/s41467-020-17224-2 | www.nature.com/naturecommunications 3 ARTICLE NATURE COMMUNICATIONS | https://doi.org/10.1038/s41467-020-17224-2 the control of the C–CandC–O bond formation (entries 2–4, groups at an early synthetic stage to limit the number of synthetic Table 1).
Details
-
File Typepdf
-
Upload Time-
-
Content LanguagesEnglish
-
Upload UserAnonymous/Not logged-in
-
File Pages9 Page
-
File Size-