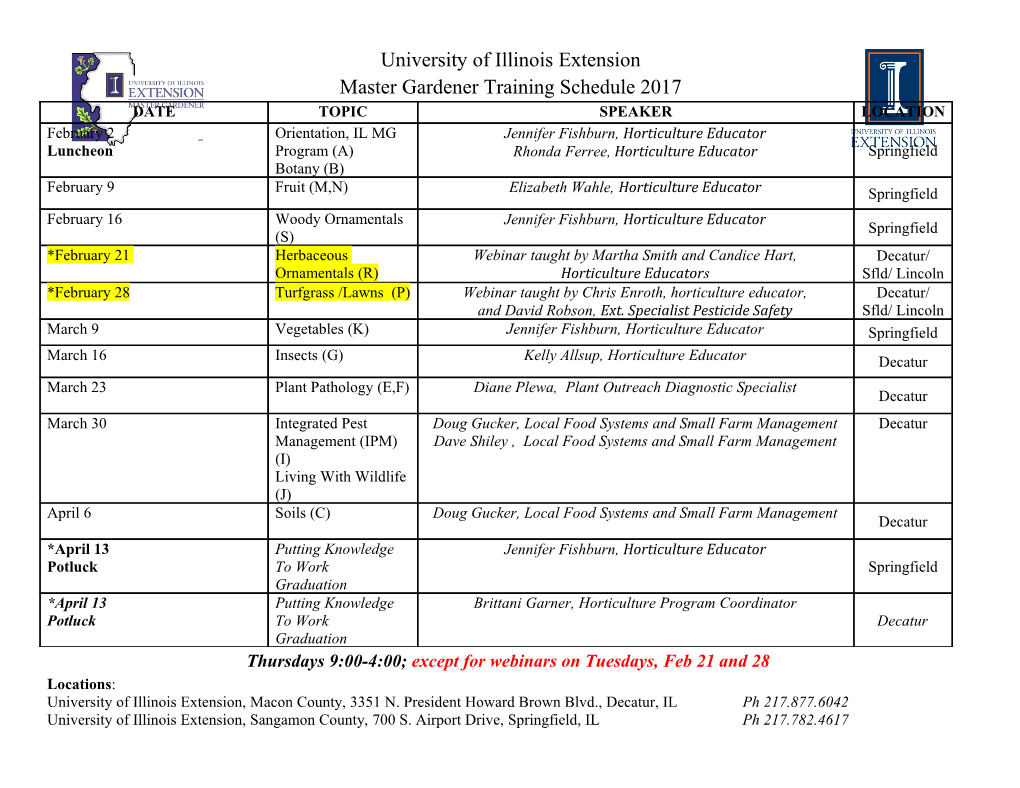
The hilernationd J014r?ifllqf Na~iticalArclineologj~ ( 1997) 26.1: 3-16 The Mary Rose site-geophysical evidence for palaeo-scour marks R. Quinn, J. M. Bull, J. K. Dix* Deprirtnieiit of Geology, *Departnient of Oceanography, Southainpton Oceaiiographv Centre, University of Sozrtlianpon, Etnpress Dock. Soutliunipton SO14 3ZH, UK J. R. Adams Department of Archneology, University of Soirtkcimpton, Higllfield SO1 7 1BJ, UK Introduction co-ordinated surveys. This trend is also Since the development-led boom in rescue reflected in the UK Government’s inter- archaeology in the 1960s and 1970s, the pretation of current legislation, in particu- vicissitudes of funding and the growing lar with respect to historic wrecks, as well recognition of the non-renewable nature as dove-tailing with the recently estab- of the archaeological resource, have lished database for maritime sites at the prompted much more circumspect atti- National Monuments Record Centre at tudes to excavation (Cleere, 1989; Hunter Swindon, UK. & Ralston, 1993). Hence the justification Over the past 30 years, various marine of decisions to excavate are generally based seismic reflection techniques have been either on the threat from development or used in the investigation of sites of other agency, or the need for research, archaeological interest, (McGhee et al., where excavation is viewed as the best or 1968; Frey, 1971; Chauhan & Almeida, only way to answer certain questions, or 1988; Rao, 1988; Redknap, 1990). To date, occasionally both. As a result, greater the application of sub-bottom profiling emphasis is now based on desk-top assess- systems to maritime archaeology has ments, non-intrusive evaluations such as been restricted by poor resolution and an fieldwalking and geophysical surveys, and inability to image the seabed in very on the associated task of building Sites’ shallow water depths. Recent advances in and Monuments’ Records (SMRs) to both acquisition and processing techniques better quantify and manage the resource. have culminated in the development of Over the same period archaeology a system known as ‘Chirp’, a digital, underwater focused on the development frequency-modulated (FM) sub-bottom of appropriate excavation and recording profiling system. methods and the collection of data. Since The principal objectives of this paper are the mid 1980s, this area of research has to present the results and associated inter- moved in a broadly similar direction, pretation of a three-dimensional Chirp concentrating less on the excavation of sub-bottom survey of the excavated Mary single sites (often as a reaction to chance Rose site carried out in conjunction with discoveries) and more on assessment and the Mary Rose Trust, and discuss the recording. An increasing number of application of Chirp technology to mari- projects in coastal regions are more pro- time archaeology. In order to familiarize active in character, involving regionally the reader with the principles of operation 1057-2414/97/010003 + 14 $25.00/0 na970060 1997 The Nautical Archaeology Society NAUTICAL ARCHAEOLOGY, 26.1 I I Transmitted ray Figure 1. Reflection and transmission from a boundary in the sub-surface. A boundary giving rise to a reflection will be marked by an acoustic impedance contrast across it. The incident ray is partitioned into a reflected ray and a transmitted ray. The proportion of incident amplitude that is reflected is controlled by the reflection coefficient. See text and Equation 1 for more details. of the sub-bottom profiling system, the of the reflected signals. Assuming a vel- following two sections concentrate on ocity at which the acoustic signal propa- seismic reflection theory and introduce the gates through the sub-bottom sediments, it aspects of Chirp sonar which set it apart is possible to estimate the depth of reflec- from conventional sub-bottom profiling tors. In seismic reflection theory, a material technology. For those interested, more is characterized by its compressional wave detailed discussions on the principles of velocity (V,) and density (p). The product acoustics can be found in many general pV, is known as the acoustic impedance. geophysics texts (including Anstey, 1981; If a contrast exists between the acoustic and Sheriff & Geldart, 1995), and the impedance of two materials (plVpl and systematics of Chirp design and operation p2V,,), then a reflection occurs at the is dealt with more comprehensively in boundary of the media (Fig. 1). The Schock & LeBlanc (1990) and Parent & strength of this reflection is governed by O'Brien (1993). Furthermore, a Notes the reflection coefficient, K,, where: section is provided at the end of the paper providing definitions for some of the KR = p2vp2 - plvpl (Equation 1) terminology and concepts introduced. PJp2+ PJ,, This equation means that the higher the The seismic reflection method contrast between the acoustic impedances The seismic reflection method utilizes the of the media, the stronger the reflection propagation of waves through the earth. from the boundary. Values of K, range Seismic reflection profiling is accomplished between - 1 and + 1. If KR= 0, then the by transmitting an acoustic signal through incident compressional wave is entirely the water column into the underlying sedi- transmitted, and no reflection occurs. Con- ments and measuring the time interval versely, if K,= - 1 or + 1 then complete between pulse transmission and the arrival reflection of the incident wave occurs, 4 R. QUINN ET AL.: THE MARY ROSE SITE - 0 32 Time (ms) Figure 2. An example of a 32 ms frequency-modulated Chirp pulse, sweeping between 2 and 8 kHz (reproduced courtesy of GeoAcoustics Limited, Great Yarmouth, UK). Note the frequency content increases to the right. and no energy is transmitted across the ics is the advent of powerful and affordable boundary. digital electronics and desktop computing In geological terms, values of K, tend to facilities. Chirp technology utilizes these fall in the range of h0.1 (Anstey, 1981) developments to aid processing and acqui- with the majority of the energy being trans- sition techniques to produce high quality, mitted. Where anthropogenic materials high-resolution sub-bottom images in real such as wood are brought into contact time. The most obvious aspects of Chirp with unconsolidated marine sediments, sonar that set it apart from conventional values of K, tend to have a broader range systems are the increased vertical resolu- (Quinn et al., 1996). Historically, wooden tion"] of Chirp systems, pulse repeatability wrecks are composed of oak, with lesser and the acquisition of data with a high components of pine. mahogany and elm. signal-to-noise ratio"] (SNR). Quinn et al. (1996) demonstrated that Unlike conventional short-pulse, single- theoretical reflection coefficients between frequency systems (e.g. pingers and oak and unconsolidated marine sediments boomers), the Chirp sonar transmits a range between - 0.03 and - 0.63 (values linearly swept, frequency modulated (FM) considerably higher than those found in pulse, i.e. the frequency of the pulse normal sub-surface geological situations) changes linearly with time (Fig. 2). In indicating a high probability of imaging conventional sub-bottom profilers, a trade- wooden artefacts using an appropriate off occurs between penetration and vertical sub-bottom profiling system. resolution. A high frequency source produces high resolution images of the Chirp technology sub-bottom, but penetration is limited. Perhaps the most important recent devel- Conversely, low frequency pulses penetrate opment in high-resolution marine geophys- deeper, but produce less-detailed sections. 5 NAUTICAL ARCHAEOLOGY, 26.1 Pinger’s typically operate in a frequency signal or noise energy does not match the range of 3 to 12 kHz, corresponding to outgoing Chirp pulse, the matched filter vertical resolutions of the order 20 cm. The attenuates the unwanted signals thus pinger’s depth of penetration is very lim- improving the SNR of the acquired data. ited however, typically tens of metres in fine muds and only several metres in coarse The Mary Rose site sediments. Boomers, with a higher energy The Mary Rose, King Henry VIII’s flag- output, offer higher depths of penetration ship, was built in 1509 and subsequently but vertical resolution is typically limited wrecked on 19 July, 1545. The wreck was to 0.5 to 1 m. The Chirp systems wide rediscovered in the Solent during the late bandwidth (typically of the order 10 kHz) 1960s (Fig. 3), and site excavation culmi- limits this trade-off between penetration nated with the raising of the hull remains and vertical resolution by transmitting a in 1982. Events leading to the location, range of frequencies, thus ensuring opti- excavation and subsequent raising of the mum penetration aid resolution. The Mary Rose are well documented (McKee, vertical resolution of the Chirp system is a 1982; Rule, 1982; Dobbs 1995). Today, function of the bandwidth of the trans- the raised hull structure is on display in mitted pulse, whereas the resolution of Portsmouth Dockyard, while the Mi4r-v conventional systems in entirely dependent Rose site itself remains one of 42 sites on the pulse length. Therefore relatively currently designated under the Protection low frequency wideband Chirp pulses can of Wrecks Act, 1973 (Archaeological be used to achieve high vertical resolution. Diving Unit, 1996). Elements of the wreck For example, a Chirp system bandwidth of remain buried on the seabed and the site is 10 kHz corresponds to a theoretical verti- constantly monitored by the Mary Rose cal resolution of 7.5 cm, assuming a com- Trust. pressional wave velocity of 1500 ms ~ ’ Prior to the positive identification of the (typical velocity value for the water wreck in the late 1960s, a number of geo- column). physical surveys were conducted over the With conventional systems, variations in supposed wreck-site in an attempt to locate the amplitude and phase of the transmitted the exact position of the Mary Rose (Rule, pulse lead to a problem in source repeat- 1982).
Details
-
File Typepdf
-
Upload Time-
-
Content LanguagesEnglish
-
Upload UserAnonymous/Not logged-in
-
File Pages14 Page
-
File Size-