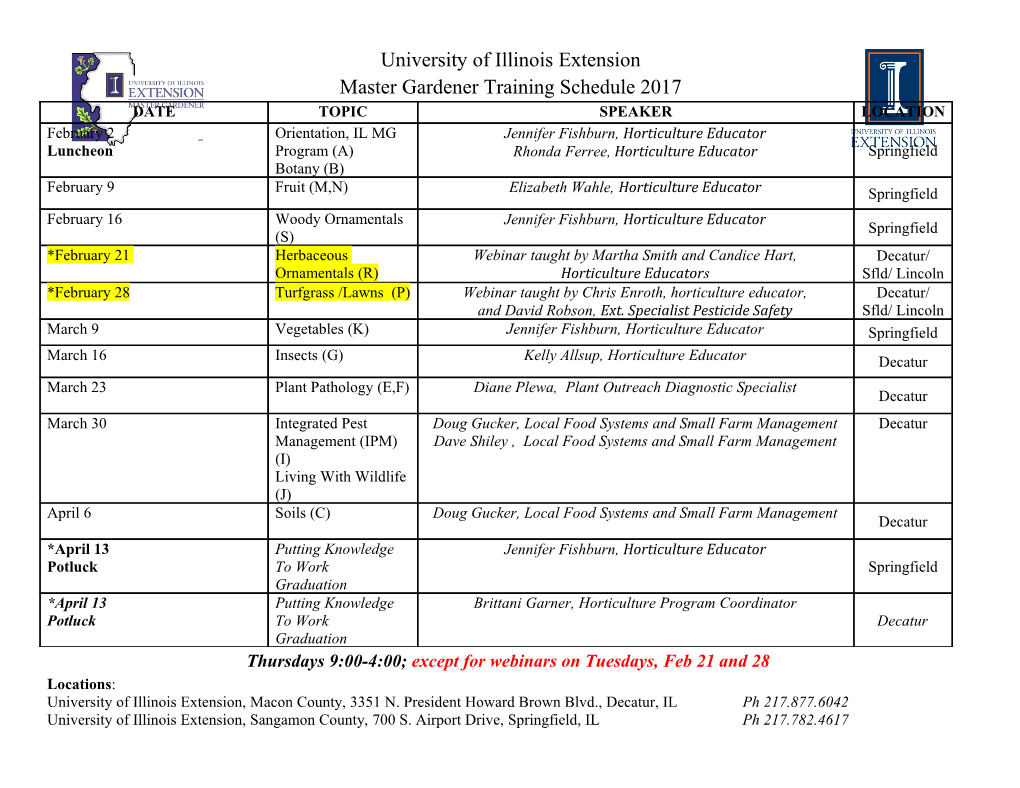
STATE OF THE ART REVIEW: FUEL CELL TECHNOLOGIES IN THE DOMESTIC BUILT ENVIRONMENT 1Theo Elmer, 2Saffa B Riffat Institute of Sustainable Energy Technology, School of Architecture and Built Environment, University of Nottingham, NG7 2RD, UK [email protected], [email protected] ABSTRACT The paper provides a state-of-the-art review of fuel cells operating in the domestic built environment. Fuel cells produce heat when generating electricity, thus they are of particular interest for combined heat and power (CHP) applications. As this paper’s focus is the domestic built environment, only micro-CHP applications are considered. The review commences with an examination of micro-CHP in the built environment and then appraises domestic fuel cell technology. It concludes with an assessment of the present development of, and future challenges for, domestic fuel cells; specifically the operational advantages they offer compared to conventional micro-CHP technologies. As fuel cells are an emergent technology the paper draws on a breadth of literature, data and experience, mostly from the UK, Japan, USA and Australia. 1. INTRODUCTION It is now of global importance that greenhouse gas (GHG) emissions associated with energy production are substantially reduced in order to limit the effects of climate change. Agreements such as the 1997 Kyoto Protocol have been established in order to try and mitigate the effects of climate change by reducing the quantities of GHGs released into the atmosphere. More recently the UK set out in its 2007 Energy White Paper it would commit to an 80% GHG emission reduction compared to 1990 levels by 2050 (DECC 2008). The UK’s 2050 target is ambitious; however there is now a common trend amongst many countries towards aspirations of a low carbon future. In the 4th Inter-governmental Panel on Climate Change Assessment the built environment was identified as holding the largest economic potential for the reduction of CO2 emissions (Hawkes, Staffell et al. 2009). Currently in the UK, over 30% of CO2 emissions are associated with the domestic housing sector. This illustrates the critical importance of decarbonising the domestic built environment if substantial CO2 emissions reductions are to be realised. Fuel cells have been identified as one of the promising technological options on the route to a zero carbon built environment (Hawkes, Staffell et al. 2009). Fuel cells are electrochemical devices which convert hydrogen and oxygen into water and in the process produce electricity. Invented in 1839, fuel cell technology is by no means new. However, in the past, fuel cells have struggled to flourish particularly in terms of commercialisation and market application. This stalled start has occurred for a variety of reasons; technological reliability, lack of interest, lack of infrastructure but mainly cost (Gencoglu and Ural 2009). On the other hand, fuel cells have been used in bespoke projects. NASA used fuel cells in some of their early space shuttles to provide electrical power, heat and water. In more recent years fuel cells have started receiving much more attention with their use in the current major market application of automobiles, with cars such as the Honda FCX Clarity (Wu and Wang 2006). However as a result of growing concerns of climate change, ever increasing cost and scarcity of fuel resources fuel cells have recently been identified as a key technological option for improving both building energy efficiency and reducing emissions (Kazempoor, Dorer et al. 2011). Fuel cells are an attractive option for building applications because of their; high electrical efficiency (even at part load), low emissions, near silent operation, flexibility of fuel use and useful heat output. Owing to the variety of fuel cells on the market (PEMFC, SOFC etc.) and their modularity, fuel cells have the ability to cover a range of building applications 1 from single family homes to an entire hospital (Kazempoor, Dorer et al. 2011). CFCL (Ceramic Fuel Cell Ltd, Australia) reported that the residential SOFC market is around 17,000 kWe installed per annum, a large market potential. E.ON believes most UK homes are technically suitable for fuel cell micro-CHP, equal to a potential total installed capacity of 24GWe (Harrison 2012). In CHP applications the heat output of the fuel cell can be used for the heating of water for domestic hot water (DHW) or space heating. Similarly combined cooling heat and power (CCHP) applications most commonly utilise the heat output in a heat driven cooling cycle such as vapour absorption, adsorption or desiccant (Wu and Wang 2006). Fuel cells are also of special interest in the built environment on account of their promise as a decentralised generator (DG). Fuel cells can provide both heat and power from a single fuel source at the end-point of use (Wu and Wang 2006). DG applications are of increasing interest because of; reduced electrical transmission losses, protection against attacks or damages to centralised plants, increased energy security and the option to utilise the commonly wasted heat from the power production process, therefore increasing overall efficiencies. Fuel cells have been trialled at a variety of scales in CHP and CCHP configurations (Gigliucci, Petruzzi et al. 2004; Pilatowsky, Romero et al. 2007; Hawkes, Staffell et al. 2009; Al-Sulaiman, Dincer et al. 2010; Calise 2011). However fuel cells in such applications are still not considered a mature technology with many technical, performance and operational uncertainties requiring further investigation (Kazempoor, Dorer et al. 2011). EneFarm field trials of 1kWe fuel cells in Japanese households has illustrated annual CO2 emission reductions of 750-1250 kg per annum, demonstrating the potential fuel cells have in assisting decarbonisation of the domestic built environment (Carter 2012). If 5.6 million homes were to install micro- CHP fuel cell technology the emission savings would be equivalent to the emissions of eight 750MW combined cycle gas turbine (CCGT) power stations (UKHFCA 2012). 2. MICRO-CHP IN THE BUILT ENVIRONMENT CHP is defined as the generation of heat and power from a single fuel source, with the view to using both products. CHP system applications can range in size from large multi MW centralised power applications to a single kW domestic home. Domestic micro-CHP is defined by the Carbon Trust as any system size up to 3 kWe. Hawkes, Staffell et al. (2009) define micro-CHP as any system between ≤3 kWe and ≤20 kWth. It is suitable to define both the thermal and power output because if the thermal output of the CHP system is greater than the demands of the building it will severely limit the operating period of the CHP system, resulting in lower efficiencies, greater emissions and longer pay back periods. As Beaussoleil-Morrison (2008) states, if the thermal output of the CHP device cannot be fully utilised, then the CHP system cannot expect to deliver a net benefit relative to grid electricity and a highly efficient condensing boiler. Therefore accurate energy load assessments and sizing of the CHP unit is essential. 2.1 Merits of micro-CHP The use of micro-CHP operating as a DG in the domestic built environment has many advantages associated with it compared to traditional centralised energy generation. These include; . Improved system efficiency, otherwise wasted heat is utilised (heating or cooling), therefore system efficiency can be elevated from as low as 30-50% in central power stations to around 70-85% in micro-CHP. Figure 1 illustrates the energy savings possible when switching from centralised energy generation to decentralised micro-CHP. Decentralised micro-CHP eliminates transmission losses which account for 6 – 24% in the EU transmission network (Peht 2006). Improved system efficiency and greater fuel utilisation leads to reduced operating costs and CO2 emissions. Electricity is regarded as having an economic value of roughly three times that of gas. Therefore converting lower cost gas to electricity allows households to recover cost and reduce energy bills. This is an important factor in the fight against fuel poverty (Staffell 2009). 2 . Centralised decarbonisation of electricity generation in many countries is problematic on account of opposition to both renewables and nuclear. Micro-CHP in consumers’ homes offers an option to assist in both decarbonising electricity production and providing energy saving benefits directly to the home owner (Staffell 2009). Figure 1 Sankey diagram of CHP system efficiency vs. conventional energy generation (Brett 2007) 2.2 Micro-CHP technologies Currently there are three main technologies used as micro-CHP generators in the domestic built environment, all at varying levels of commercial and technological maturity; Internal combustion engine (ICE), Stirling engine (SE) and fuel cells. The performance and operational characteristics of these three technologies are summarised in Table 1. It is evident that fuel cells have some clear operational advantages, particularly when operating in the built environment. These include; higher electrical efficiencies, low heat to power ratios (H:P) and near silent operation. However, the relative infancy of fuel cells limits their extensive application and market involvement. Table 1 Domestic micro-CHP technologies (Wu and Wang 2006; Hawkes, Staffell et al. 2009; Staffell 2009) Internal Combustion Engine Stirling Engine Fuel Cell Capacity (electrical) 1kW+ 1kW+ 1kW + Electrical efficiency 20 10 35 - 60 Overall efficiency 85 90 85 Heat to Power ratio 3 : 1 10 : 1 1 : 1 Able to vary output No No Yes Fuel used Gas, biogas, liquid fuels Gas, biogas, butane Hydrocarbon fuel Noise Loud Fair Quiet Maturity High Fair Low Companies producing Baxi Whispergen Baxi, Bluegen (CFCL) The H:P of a CHP unit is the quantity of heat energy produced per unit of electricity generated and is an important system characteristic. Conventional CHP systems are sized to thermal loads and are only operational when the demand for energy is both electrical and thermal i.e.
Details
-
File Typepdf
-
Upload Time-
-
Content LanguagesEnglish
-
Upload UserAnonymous/Not logged-in
-
File Pages17 Page
-
File Size-