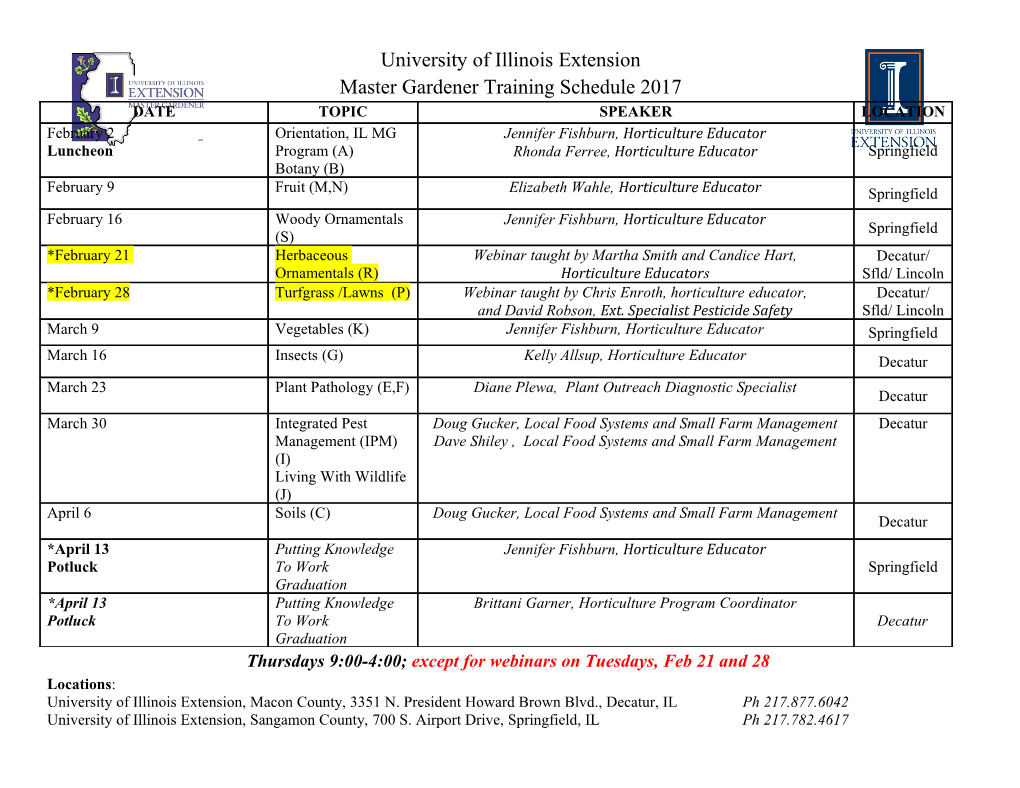
EFFECTS OF PULSED LOW FREQUENCY ELECTROMAGNETIC FIELDS ON WATER CHARACTERIZED BY LIGHT SCATTERING TECHNIQUES: ROLE OF BUBBLES Philippe Vallée1*, Jacques Lafait1, Laurent Legrand2, Pascale Mentré3, Marie-Odile Monod4, Yolène Thomas5 1 Laboratoire d’ Optique des Solides (UMR CNRS 7601) Université Pierre et Marie Curie, Campus Boucicaut 140, rue de Lourmel, 75015 Paris, France 2 Groupe de Physique des Solides (UMR CNRS 7588) Universités Pierre et Marie Curie (Paris 6) et Denis Diderot (Paris 7) Campus Boucicaut 140, rue de Lourmel, 75015 Paris, France 3 UMR 8646 - MNHN 53 rue Buffon, 75005 PARIS, France 4 CEMAGREF 24, avenue des Landais - BP 50085 63172 AUBIERE Cedex, France 5 Institut Andre Lwoff IFR89 7, rue Guy Moquet-BP8 94801 Villejuif Cedex, France *Corresponding author: [email protected] Langmuir 20005, in press Abstract Well-characterized purified water was exposed for 6 h to pulsed low-frequency weak electromagnetic fields. After various time periods, nondegassed and degassed water samples were analyzed by static light scattering. Just after electromagnetic exposure (day 0), a reduction of over 20% in the maximum light scattering intensity at 488 nm wavelength in both nondegassed and degassed samples was observed. By contrast, on day 12 the difference was observed only in nondegassed water samples. The latter effect was attributed to the different geometries of the containers combined with the basic origin of the whole phenomenon due to gas bubbles present in water. By the use of dynamic light scattering, the bubble mean diameter was estimated to be around 300 nm. Our results suggest that the electromagnetic exposure acts on gas nanobubbles present in water and emphasizes the role of the gas/liquid interface. The possibility that exposure to electromagnetic fields disturbs the ionic double-layer that contributes to bubble stabilization in water is discussed. Keywords: Water, atmospheric environment, electromagnetic fields, light scattering, bubbles, gas/water interface. 1 Introduction During the past decade, there has been considerable investigation of the effects of static magnetic and electromagnetic fields (EMF) on water or on the behavior of aqueous solutions and suspensions1-4. The physicochemical properties of water (oxidation-reduction potential, pH, etc.) may be altered by the magnetic and electromagnetic fields4,5. These changes depend on the field intensity and/or frequency6,7. Despite intensive research, the mechanisms by which such electromagnetic fields act on water are still a controversial issue. Indeed, it has been difficult to obtain good experimental reproducibility, mainly due to variation in water composition and/or environmental conditions. Among the different hypotheses put forward regarding the targets of EMF, the colloid/water interface appears to be the most probable. For instance, Chibowski et al.8 studied the effects of EMF in the radio frequency range (44 MHz) on pH, conductivity and ζ potentials of colloidal particles of different oxides. They observed noticeable oscillations in the ζ potential for hours after treatment. Colic and Morse9, working with radio frequency EMF on colloids in water, proposed that the electromagnetic exposure effect results from a perturbation of gas/liquid interface and noted that degassing removes all of the observed effects (ζ potential, turbidity, etc). These changes persist for hours and even days10. Fesenko et al.5, treating triply distilled deionized water with microwaves, suggested that the EMF might act on gas dissolved in water. Eberlein11,12 proposed a quantum vacuum radiation explanation of the effect based on the influence of an oscillating EMF on the gas/water interface. Previous studies from our laboratory13 and others14 show that species arising from container/content interactions affect the physicochemical properties of water. We developed and present in this paper new experimental procedures to investigate the effect of pulsed low- frequency EMF on water. In particular, special attention has been paid to (1) water purification and physicochemical characterization and (2) environmental conditions (atmospheric, 2 electromagnetic, and acoustic). Moreover, glassware was made of pure fused optical silica in order to minimize the release of compounds from the containers. Experiments were also carried out to explore the influence of gas bubbles on the EMF effect by using static and dynamic light scattering. Finally, we discuss different mechanisms for the action of the electromagnetic fields, in particular via a mechanical action on the ionic charges present around gas bubbles. 1. Experimental Section 1.1 Materials Water Water used in the experiments described below was freshly prepared from Paris tap water (pressure 6 bar) after two purification steps using: (1) an inverse osmosis apparatus (Millipore, Rios III) that eliminates molecules between 0.2 and 1 nm as well as 97% of ionic substances and (2) a final purification apparatus (Millipore, SimplicityTM) equipped with an ultrafilter (Millipore, Pyrogard D, size cutoff of 13,000 Da) which eliminates the remaining organic compounds ≥ 5-10 nm. At the end of the procedure, the purified water was apyrogenic according to the manufacturer and the resistivity was measured to be 18.2 MΩ.cm at 25 °C. Glassware All glassware was made of pure fused optical silica (Heraeus, Suprasil) in order to minimize container/content interaction13,14. Optical cells (Hellma, Qs111, of volume: 3.5 mL and cross section: 1 cm2), were closed with a pure fused silica cap. Glassware was thoroughly washed with spectroscopy grade ethanol (Merck Uvasol, purity 0.999) to remove any surface active material and then put in a clean oven at 80°C for 15 min. Just before use, the optical cells were rinsed with purified water. A special glassware apparatus made of two compartments, a round beaker connected to an optical cell, was used to degas water. 3 Glovebox To ensure a controlled atmosphere, samples were prepared inside a glovebox (Jacomex, Altuglass model B003). To reconstitute atmospheric air, argon (N56, Air Liquide), carbon dioxide (N48, Air Liquide), oxygen, and nitrogen (Air N57 Pol, Air Liquide) were filtered through a 0.2 µm filter (Osmonics, Teflon Calyx Capsule) and then introduced into the glovebox. To avoid contamination from outside, a pressure 3 mbar above ambient was maintained. Relative humidity (60 ± 2%) and carbon dioxide (310 ± 20 ppm) were measured using a Vaisala GM70 hand-held meter (Vaisala, calibrated probe, humidity: HMP75 and carbon dioxide: GMP70). Insulation cages and storage boxes To control environmental conditions during the exposure to electromagnetic fields, samples were kept in thermally, acoustically, and magnetically shielded containers (diameter 700 mm, 500 mm high). Thermal and acoustic insulation were achieved by using multilayers of various polymers (Illbruck Company, EPDM, density: 8kg/m3, 20 mm thick; melamine resin, density: 11kg/m3, 20 mm thick and bitumen mass, density 10kg/m3, 3 mm thick). For magnetic insulation we used a double layer of mu-metal (Meca-Magnetic, two foils of “Mumetal”, each 1mm thick). According to the manufacturer, the ambient magnetic field intensity (0.1 mT root mean square (rms), ac, 50 Hz) is reduced by a factor of 850 (≈ 58 dB). A Faraday cage of copper foil (2 mm thick) was used for electrical insulation. In addition, two cylindrical storage boxes made of mu-metal (diameter 80 mm, 105 mm high, 2 mm thick) were built to shield the closed samples. Each storage cylinder was finally put into a Thermos® box. 4 1.2 Water treatments Electromagnetic exposure Optical cells containing purified water were placed in one of the two insulation cages and exposed for 6 h to pulsed (duration 30 s) electromagnetic fields generated by a solenoid coil (diameter 50 mm, 80 mm high, copper wire, 4367 turns/m, self-inductance L = 3 mH, ohmic resistance 3 Ω). The electric signal applied to the coil, provided by a programmable function generator (Agilent 33120A), was composed of three successive sinusoidal pulse trains in the frequency range from 10 to 500 Hz. Optical cells were placed in a vertical position in the center of the coil supplied by a 250 mA (rms) current. The calculated rms magnetic field density at the center of the coil is ≈ 1 mT and, according to Faraday’s law, the maximum induced electric field is 4.1 mV/m. Reference (untreated) optical cells were placed in the other insulation cage under the same conditions except they were not exposed to the electromagnetic fields. After the electromagnetic exposure, optical cells (reference (R) and treated (T)) were transferred and kept in separate storage boxes. The temperature has been measured during the electromagnetic exposure, by using a chromel/alumel thermocouple (∆T was less than 0.01 °C). Degassing process (Fig. 1) A zeolite pump was used to degas purified water (20 min, at a pressure of 1.6x103 Pa, as measured by a Comark, manometer model C9555). Degassing was done prior to exposure to electromagnetic fields in the dedicated glassware (1/7 of the total volume filled with water) as described above. After pumping, degassed water was transferred from one compartment of the glassware (Suprasil round beaker) to the connected optical cell compartment. Degassed reference water (DR) and degassed treated water (DT) samples are thus ready for optical measurements. Note that, in nondegassed samples, the optical cells were filled to the top with water and sealed with the cap. 5 1.3 Measurements Physicochemical water analysis All the measurements using a multiparametric system (Fisher Scientific, Consort C835 multimeter) were always done in the glovebox, in the same order: conductivity/temperature, oxygen content, pH, and oxidation-reduction potential (ORP). Multi-meter data were logged on a computer. For conductivity measurements the apparatus was equipped with a specific electrode of cell constant K = 0.1 cm-1 for low ionic concentration solutions with automatic temperature correction (Pt1000) and calibrated with two ionic strength solutions (Hanna Instruments, 84 and 1413 µS cm-1 at 25 °C). pH measurements were done using a combined electrode (Metrohm, LL- Aquatrode) adapted for pure water.
Details
-
File Typepdf
-
Upload Time-
-
Content LanguagesEnglish
-
Upload UserAnonymous/Not logged-in
-
File Pages27 Page
-
File Size-