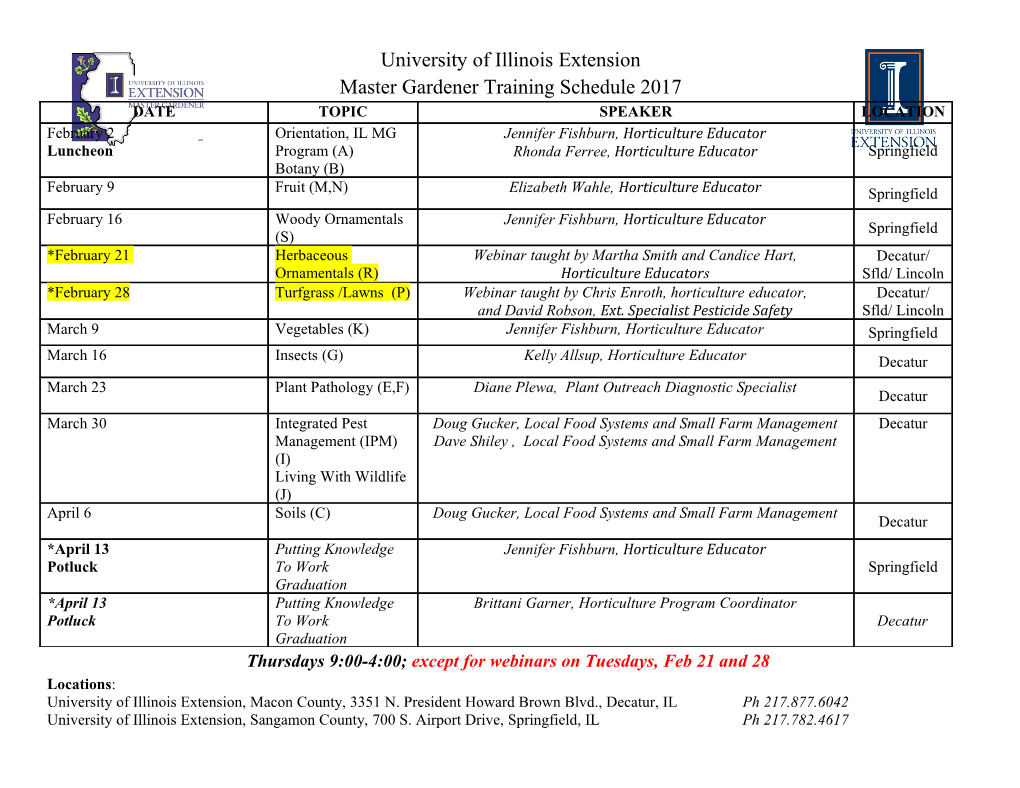
Spherical Tokamak for Economical Fusion Energy Development Masayuki Ono NSTX-U Department Head PPPL, Princeton University PPPL Innovation for Cool Earth Forum October 5 - 6, 2016 M. Ono ICEF 2016 October 5, 2016 Fusion for safe limitless energy source Fusion could provide energy for Energy 10 million times that of fossil fuel by weight future mankind: D + T He4 + n + 17.6 MeV - Environmentally friendly Heat from fusion reactor can also produce hydrogen! - Safe - Globally abundant fuel - High energy density - Support hydrogen economy Global Warming Nuclear spent fuel A large asteroid / comet hit Earh 65 million years ago Annual CO2 release – 40 billion tons Still increasing 8,000 tons per year M. Ono ICEF 2016 October 5, 2016 2 Fusion for safe limitless energy source Fusion can also solve potential challenges for humanity Fusion could provide energy for Energy 10 million times that of fossil fuel by weight future mankind: D + T He4 + n + 17.6 MeV - Environmentally friendly Heat from fusion reactor can also produce hydrogen! - Safe - Globally abundant fuel - High energy density - Support hydrogen economy Fusion could help solve future challenges facing mankind: - Global warming - Fission reactor spent fuel - Space travel Global Warming Nuclear spent fuel A large asteroid / comet hit Earh 65 million years ago Annual CO2 release – 40 billion tons Still increasing 8,000 tons per year M. Ono ICEF 2016 October 5, 2016 3 Nuclear Fusion has many possible approaches Many Types of Magnetic Bottles! Beta is a ratio of plasma pressure over magnetic pressure - Plasma pressure produces fusion power - Mangetic pressure provided by coils but cost $ Tokamak Tri-Alpha NSTX-U Energy (Private) (US-DOE) A Modern Conventional Spherical (Private) Compact Stellarator Tokamak Tokamak Toroids Very Low B Axi-symmettric High beta Ip / IC ~ 0 Ip / IC ~ 0.1 Ip / IC ~ 1 Ip / IC > 1 LHD, W7-X TFTR, JET, JT-60, ITER NSTX, MAST. MST, RFX Ip = plasma current helps plasma confinement and stability Ic = coil current also helps plasma confinement and stability but cost $ M. Ono ICEF 2016 October 5, 2016 4 Nuclear Fusion has many possible approaches Many Types of Magnetic Bottles! What is the best fusion approach? Higher beta*, smaller size, lower field, economical Tokamak Tri-Alpha NSTX-U Energy (Private) (US-DOE) A Modern Conventional Spherical (Private) Compact Stellarator Tokamak Tokamak Toroids Very Low B Axi-symmettric High beta Ip / IC ~ 0 Ip / IC ~ 0.1 Ip / IC ~ 1 Ip / IC > 1 Enable more external control *Beta is a ratio of plasma pressure over magnetic pressure M. Ono ICEF 2016 October 5, 2016 5 Princeton Plasma Physics Laboratory PPPL investigates many type of magnetic bottles and plasma applications Model A, B C-Stellarator ST, ACT, PLT, PDX, PBX TFTR NSTX NSTX-U Matterhorn1960 1970 1980 1990 2000 2010 1951 Stellarators Conventional Tokamaks 10 MW Spherical Tokamaks Princeton U I joined Fusion Fusion Lab. Atoms for the lab Peace 1958 Power TFTR Tokamak NSTX Spherical Tokamak A Modern Stellarator Conventional Tokamak Spherical Tokamak Axi-symmettric High beta M. Ono ICEF 2016 October 5, 2016 6 A spherical tokamak (ST) is a high beta tokamak Favorable average curvature improves stability at high beta 2 Aspect Ratio A = R /a Elongation κ = b/a Toroidal Beta βT = 〈p〉 / (BT0 / 2µ0) Camera image from NSTX-U Tokamak ST A ~ 3, A ~ 1.5, κ = 1.5-2, κ = 2-3, q95 = 3-4, q95 = 8-12, βT = 3-10% βT = 10-40% M. Ono ICEF 2016 October 5, 2016 7 Operating ST Research Facilities Since 2000 NSTX and MAST: MA-class STs, Smaller STs addressing topical issues NSTX, USA PEGASUS, USA LTX/CDX-U, USA GLOBUS-M, Russia MAST, UK HIST, Japan LATE, Japan TST-2, Japan UTST, Japan R0 ~ 0.75 m QUEST/CPD, Japan R0 ~ 0.85 m VEST, Korea HIT-II, USA GLOBUS-M PEGASUS QUEST HIT-II MAST z KTM HIST ST25(HTS) SUNISTVEST z NSTX/LTX LATE TST-2 TS3/4 UTST TS3/4, Japan SUNIST, China ETE, Brazil ST25, UK ETE STs operated since 2000 z M. Ono ICEF 2016 October 5, 2016 8 NSTX-U Facility Came On Line This year To demonstrate fully sustained high beta plasmas nd 2 NBI 1st NBI HHFW System NSTX-U M. Ono ICEF 2016 October 5, 2016 9 Unique ST properties support and accelerate a range of development paths toward fusion energy Extend Predictive Capability for ITER and Burning Plasma Physics - Toroidal Science ITER High β physics, rotation, shaping for MHD, transport Non-linear Alfvén modes, fast-ion dynamics, Electron gyro-scale turbulence at low ν* M. Ono ICEF 2016 October 5, 2016 10 Higher βT enables economical fusion power and compact neutron sources for near term and long term applications 2 Pfusion ∝ 〈p〉 x Vol Data base from START, MAST, PEGASUS and NSTX βN = 6 40 2 4 5 Pfusion ∝ βT BT0 x Vol 4 (%) β ST-FNSF Investment Product Physics 3 cost 20 Knob Toroidal Tokamak 2 ITER Higher β allows lower BT0 and/or smaller Vol both lower cost 0 0 4 8 Ip/aBT0 (MA/mT) Note: Ultra high β STs ~ Lower BT0 Lower Cost, Greater Safety, and 50% produced in TS-3 Reliability Device at Univ. Tokyo M. Ono ICEF 2016 October 5, 2016 11 0.0 0.2 0.4 0.6 0.8 1.0 -1 log10(disruptivity [s ]): N -0.5 -0.25 0.0 0.25 0.5 0.75 1.00 0.0 0.2 0.4 a)0.6 0.8 1.0 6 N β 4 NSTX Data Demonstrates a Favorable Operations Window At 2 High β For Reduced Disruptivity in an ST-FNSF -1 Good!35237log 10total(disruptivity samples [s ]): 2 3 4 5 6 N -0.5 -0.25 0.0 q*0.25 0.5 0.75 1.00 a) STb) 6 reactors 6 S=37 S=20 N β 4 N β 4 2 2 35237 total samples 1.5 2.0 2.5 3.0 3.5 4.0 35237 total samplesp0/<p> • No strong increase in disruptivity as βN increases 10 20 30 40b) S • Reduction in disruptivity also with: 6 (S=q95IP/aBT) • Decreasing li (broader current profile) Example: Disruptivity is reduced • Decreasing pressure peaking N β 4with strong shaping of the Upgrades will test and improve these plasma boundary. favorable trends in a systematic way 2 S.P. Gerhardt et al., NF (2013) 35237 total samples M. Ono ICEF 2016 October 5, 2016 12 0.4 0.6 0.8 1.0 1.2 li Understanding Electron Energy Confinement is Critical for Reactor Design Energy Diffusivity or Rate of Energy Loss Electron energy is NSTX escaping from fusion plasma core much faster (much more than 10x) than neoclassical prediction – anomalous! Ion energy is escaping from fusion plasma core not much faster than neoclassical prediction • Fusion Alpha-particle energy mainly heats electrons • If electron energy loss is rapid, alpha-heating cannot keep fusion going Y. Ren. M. Ono ICEF 2016 October 5, 2016 13 Encouraging Confinement Trend with Collisionality in STs Important implications for future STs if trend continues NSTX MAST Increasing confinement Increasing electron temperature Increasing electron temperature S.M. Kaye et al., NF(2013) M. Valovic et al., NF (2011) M. Ono ICEF 2016 October 5, 2016 14 Favorable confinement trends with Te and β help make economical compact fusion reactors Fusion triple product = n τ T • Conventional energy confinement time τ improves with device size (or volume) and magnetic field. But the cost increases with V and B. • ST scaling suggests confinement improvement can be achieved with increased temperature which is Hot achieved in reactor naturally for Plasma Core free. • Improved confinement could lead to smaller volume more economical fusion reactor • Better confinement could also enable small neutron sources which have many applications NSTX-U Micro-tearing simulation by W. Guttenfelder and F. Scotti M. Ono ICEF 2016 October 5, 2016 15 Favorable confinement trend with collisionality and β found in ST experiments -0.8 -0.0 ST scaling observed in NSTX and MAST: τE, th ∝ ν*e β -0.1 -0.9 Tokamak empirical scaling (ITER 98y,2): τE, th ∝ ν*e β Surprising confinement scaling in STs! Confinement time actually increased with temperature while previous scaling Lithium predicted just the Reactor Boron opposite! regimes Increasing Te Also no confinement degradation observed in ST with plasma β M. Ono ICEF 2016 October 5, 2016 16 Several ST Fusion Power Plants Design Studied Low Toroidal Field and Magnetic Energy ARIES-ST JUST Cu Power Plant SC ST Power Plant R0 ~ 3.2 m R0 ~ 4.5m BT0 ~ 2.1T BT0 ~ 2.36T F. Najmabadi et al., FED (2003) Y. Nagayama et al., IEEJ (2012) H. R. Wilson, et al., NF (2004) B.G. Hong, Yet al.,NF (2011) K. Gi NF (2015) 17 M. Ono ICEF 2016 October 5, 2016 ST Fusion Plant Designed With High Temperature SC A=2, R0 = 3m HTS-TF FNSF / Pilot Plant BT = 4T, IP = 12.5MA κ = 2.5, δ = 0.55 βN = 4.2, βT = 9% H98 = 1.8, HPetty-08 = 1.3 fgw = 0.80, fBS = 0.76 Startup IP (OH) ~ 2MA 2 JWP = 70MA/m BT-max = 17.5T No joints in TF Vertical maintenance Pfusion = 520 MW PNBI = 50 MW, ENBI = 0.5MeV QDT = 10.4 Qeng = 1.35 Pnet = 73 MW 2 〈Wn〉 = 1.3 MW/m Peak n-flux = 2.4 MW/m2 Peak n-fluence = 7 MWy/m2 J. Menard et al., NF (2016) by PPPL, …Tokamak Energy M. Ono ICEF 2016 October 5, 2016 18 Fusion to Solve Fusion Waste Material Problem Only a Compact Fusion ST Cores Needed For Neutron Production Tritium ST Fusion-Fission Hybrid Lithium ST Heat, Electricity 50 – 100 MW Fusion Fission 1 – 3 GWt Core Spent Fuel 100 MW 14 MeV Fission Fuel Fusion neutrons World is full of spent fuel from fission reactors – 20 t per year per reactor – 76,000 t just in the USA • High energy fusion neutrons can break down the spent fuel further to reduce long live isotopes from fission spent fuel, e.g., Pu242, Am243, Cm244 and Cm246.
Details
-
File Typepdf
-
Upload Time-
-
Content LanguagesEnglish
-
Upload UserAnonymous/Not logged-in
-
File Pages21 Page
-
File Size-