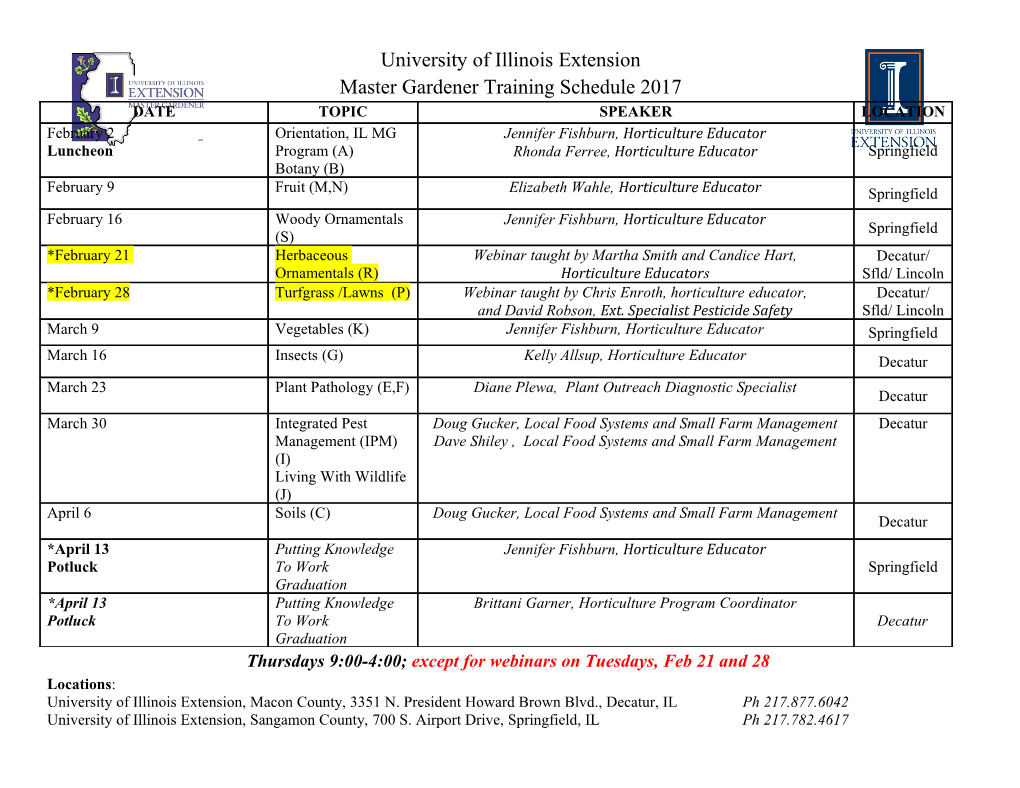
bioRxiv preprint doi: https://doi.org/10.1101/2020.10.07.329532; this version posted October 7, 2020. The copyright holder for this preprint (which was not certified by peer review) is the author/funder, who has granted bioRxiv a license to display the preprint in perpetuity. It is made available under aCC-BY 4.0 International license. 1 1 Characterisation of Metarhizium majus (Hypocreales: 2 Clavicipitaceae) isolated from the Western Cape province, 3 South Africa 4 5 Letodi L. Mathulwe1, Karin Jacobs2, Antoinette P. Malan1*, Klaus Birkhofer3, Matthew F. 6 Addison1, Pia Addison1 7 8 1 Department of Conservation Ecology and Entomology, Faculty of AgriSciences, Private 9 Bag X1, Matieland 7602, Stellenbosch, South Africa 10 2 Department of Microbiology, Faculty of Science, Private Bag X1, Matieland 7602, 11 Stellenbosch, 7602, South Africa 12 3 Department of Ecology, Brandenburg University of Technology, Cottbus, Germany 13 14 15 *Corresponding author 16 E-mail: [email protected] (APM) 17 bioRxiv preprint doi: https://doi.org/10.1101/2020.10.07.329532; this version posted October 7, 2020. The copyright holder for this preprint (which was not certified by peer review) is the author/funder, who has granted bioRxiv a license to display the preprint in perpetuity. It is made available under aCC-BY 4.0 International license. 2 18 Abstract 19 Entomopathogenic fungi (EPF) are important soil-dwelling entomopathogens, which can be 20 used as biocontrol agents against pest insects. During a survey of the orchard soil at an organic 21 farm, the EPF were identified to species level, using both morphological and molecular 22 techniques. The EPF were trapped from soil samples, taken from an apricot orchard, which 23 were baited in the laboratory, using susceptible host insects. The identification of Metarhizium 24 majus from South African soil, using both morphological and molecular techniques, is verified. 25 The occurrence of M. majus in the South African soil environment had not previously been 26 reported. 27 28 Keywords: Entomopathogenic fungi; Metarhizium majus; Morphological; Molecular; 29 Biological control bioRxiv preprint doi: https://doi.org/10.1101/2020.10.07.329532; this version posted October 7, 2020. The copyright holder for this preprint (which was not certified by peer review) is the author/funder, who has granted bioRxiv a license to display the preprint in perpetuity. It is made available under aCC-BY 4.0 International license. 3 31 Introduction 32 Entomopathogenic fungi (EPF), which are cosmopolitan components of the soil microbiota, 33 are commonly isolated from the soil environment, for use as biological control agents to 34 manage a broad range of pest insects [1,2]. The genus Metarhizium Sorokin (Ascomycetes, 35 Hypocreales) consists of asexually reproducing EPF species, which are characterised by the 36 production of green conidia on the surfaces of infected insect cadavers, and when they are 37 grown on a growth medium [3]. Species belonging to the genus Metarhizium are well-studied 38 entomopathogens, which are widely commercialised. Many products derived from the species 39 are on the market, for use against a wide range of economically-important insect pests [4,5]. 40 Distinguishing between different Metarhizium species morphologically is based on their 41 conidial morphology, as using other morphological characteristics is challenging [3]. 42 Metarhizium species are mainly identified, and differentiated from each other, using molecular 43 techniques [6]. Two main monophyletic groups fall within the Metarhizium anisopliae species 44 complex. The PARB clade consists of Metarhizium pinghaense Chen & Guo, Metarhizium 45 anisopliae sensu stricto, Metarhizium robertsii (Metchnikoff) Sorokin and Metarhizium 46 brunneum Petch, while the MGT clade consists of Metarhizium majus Johnst., Bisch., Rehner 47 and Humber and Metarhizium guizhouense Chen and Guo [3,7]. The MGT species are 48 distinguished from the PARB clade by their relatively large conidia, with M. majus having the 49 larger cylindrical conidia relative to M. guizhouense, which possess the second largest conidia 50 [7]. Metarhizium majus and M. guizhouense have been differentiated from each other, based 51 on molecular data, using the translation elongation factor-1 alpha gene (EF-1α) [3,7]. 52 In the current study, additional regarding the morphological and molecular evidence is 53 provided to present the first report on the occurrence of Metarhizium majus in South African 54 soil. bioRxiv preprint doi: https://doi.org/10.1101/2020.10.07.329532; this version posted October 7, 2020. The copyright holder for this preprint (which was not certified by peer review) is the author/funder, who has granted bioRxiv a license to display the preprint in perpetuity. It is made available under aCC-BY 4.0 International license. 4 55 Materials and Methods 56 Collection of soil samples and EPF baiting 57 Soil samples were collected from the orchards surveyed, at a depth of 15 cm, from under the 58 tree canopy on Tierhoek farm (-33˚13'19.687''S 19˚38'44.281'') (-33,711596; 19,790730), near 59 Robertson in the Western Cape province. After an initial sifting, each soil sample was 60 transferred to 1-L plastic containers, baited with the last-instar larvae of the wax moth Galleria 61 mellonella L. (Lepidoptera: Pyralidae) and Tenebrio molitor (Coleoptera: Tenebrionidae), kept 62 for 14 days at a room temperature of 25ºC [8,9,10]. The soil samples were everted after every 63 3 days, so as to ensure the penetration of the insect bait into the soil. After every 7 days, the 64 dead insects that showed EPF infection, which was observed in the form of hardening, or overt 65 mycosis of the insect cadaver, were removed from the soil samples. To check the cause of 66 mortality, the dead insects, after first being washed in sterile distilled water, were then dipped 67 in 75% ethanol, followed by them being dipped twice in distilled water. Each dead insect was 68 placed in a Petri dish fitted with moist filter paper. The Petri dishes were then placed in 2-L 69 plastic containers, fitted with paper towels moistened using sterile distilled water, and 70 incubated at room temperature. 71 Following a further 7 days of incubation, the spores from the surface of the dead insect 72 cuticles were placed on a Sabouraud dextrose agar plate with 1 g of yeast extract (SDAY), 73 supplemented with 200 µl of Penicillin-Streptomycin, so as to prevent bacterial contamination. 74 After the SDAY plates were sealed and incubated at 25ºC, they were checked for fungal growth 75 for a period of two weeks. The pathogenicity against insects was verified, using the larvae of 76 the wax moth [11]. 77 Morphological identification bioRxiv preprint doi: https://doi.org/10.1101/2020.10.07.329532; this version posted October 7, 2020. The copyright holder for this preprint (which was not certified by peer review) is the author/funder, who has granted bioRxiv a license to display the preprint in perpetuity. It is made available under aCC-BY 4.0 International license. 5 78 Temporary slides were prepared by means of trapping spores in a drop of water on a glass slide 79 with a cover slip, secured with glyceel. The size of the conidia was determined, measuring both 80 the length and the width of 30 spores, using a Zeiss Axiolab 5 light microscope, equipped with 81 an Axiocam 208 camera. SEM preparation of spores of different Metarhizium species 82 including M. majus, M. robertsii (GenBank accession number MT378171), M. pinghaense 83 (MT895630) and M. brunneum (MT380848) were prepared and photographed by the Central 84 Analytical Facility of the Stellenbosch University. 85 Molecular identification 86 For molecular identification, the fungal DNA was extracted from culture plates, using a Zymo 87 research Quick-DNA fungal/bacterial miniprep kit, according to the manufacturer’s protocol. 88 The polymerase chain reaction was conducted, using the KAPA2G ReadyMix PCR kit. 89 Characterisation was based on sequencing of the ITS region and two genes, consisting of the 90 internal transcribed spacer (ITS) (primers ITS1and ITS4), the partial tubulin (BtuB) (primers 91 Bt2a and Bt2b) and the partial EF-1α (primers EF1F and EF2R) [12,13]. The PCR thermocycle 92 conditions accorded with the technique used by [14]. The PCR products were visualised on an 93 agarose gel, using ethidium bromide. The sequences, which were generated by the Central 94 Analytical Facility at Stellenbosch University, were aligned and edited using the CLC main 95 workbench (ver. 8) and blasted on the GenBank database of the National Centre for 96 Biodiversity Information (NCBI) for identification. Fungal cultures were deposited at the 97 Agricultural Research Council (ARC), the Biosystematics Division, Pretoria fungal collection. 98 Phylogenetic analyses 99 Phylogenetic analyses were conducted using the dataset from Rehner and Kepler (2017) [13] 100 and Luz et a. (2019) [12] , combining sequences of three loci (ITS, BT, tef1), so as to identify 101 the species concerned. The alignments were done employing ClustalX, using the L-INS-I bioRxiv preprint doi: https://doi.org/10.1101/2020.10.07.329532; this version posted October 7, 2020. The copyright holder for this preprint (which was not certified by peer review) is the author/funder, who has granted bioRxiv a license to display the preprint in perpetuity. It is made available under aCC-BY 4.0 International license. 6 102 option. The software package PAUP [15] was used to construct a neighbour-joining 103 phylogenetic tree, using a bootstrap analysis of 1 000 replicates. A Bayesian analysis was run 104 using MrBayes ver. 3.2.6 [16]. The analysis included four parallel runs of 200 000 generations, 105 with a sampling frequency of 200 generations. The posterior probability values were calculated 106 after the initial 25% of the trees were discarded.
Details
-
File Typepdf
-
Upload Time-
-
Content LanguagesEnglish
-
Upload UserAnonymous/Not logged-in
-
File Pages17 Page
-
File Size-