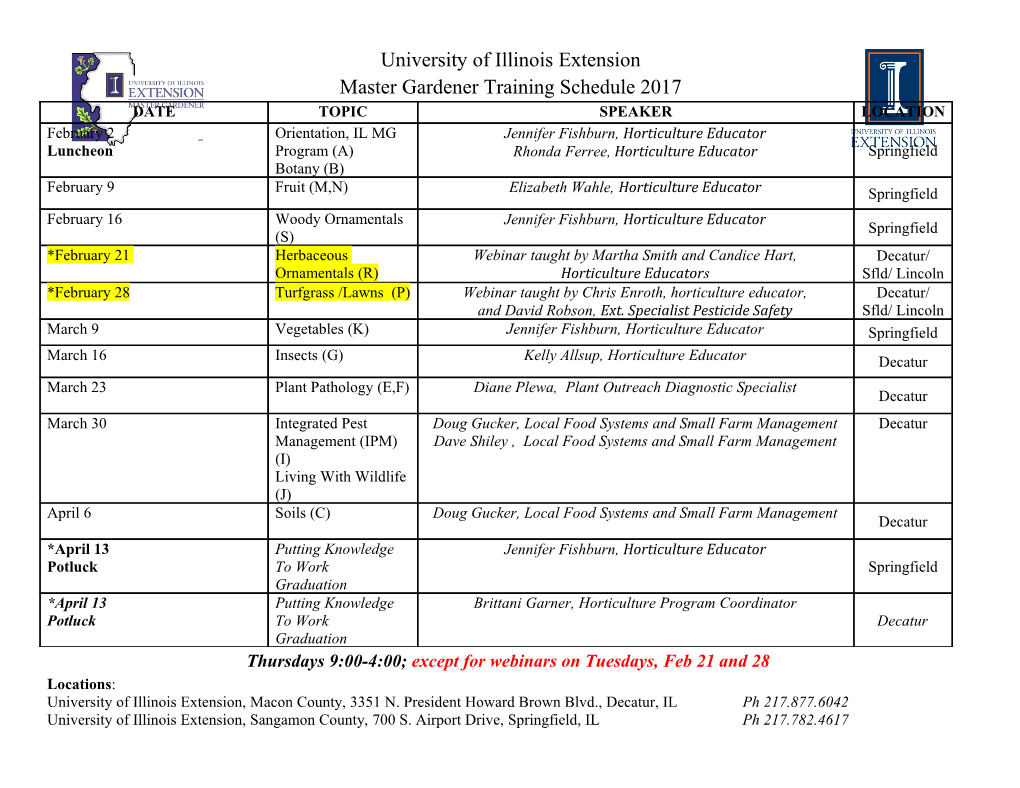
Cyclobutanone analogues of ‐lactam antibiotics: ‐lactamase inhibitors with untapped potential? Prarthana Devi and Peter J. Rutledge* Dr Prarthana Devi, Prof. Dr. P. J. Rutledge, School of Chemistry, The University of Sydney, Sydney, NSW 2006 Australia, E‐mail: [email protected]; Tel.: +61 2 9351 5020; Fax: +61 2 9351 3329. Abstract ‐Lactam antibiotics have been used for many years to treat bacterial infections. However the effective treatment of an increasing range of microbial infections is threatened by bacterial resistance to ‐lactams: the prolonged, widespread and at times reckless use of these drugs has spawned widespread resistance, which renders them ineffective against many bacterial strains. The cyclobutanone ring system is isosteric with ‐lactam: in cyclobutanone analogues, the eponymous cyclic amide is replaced with an all‐carbon ring, the amide N substituted by a tertiary C–H to a ketone. Cyclobutanone analogues of various ‐lactam antibiotics have been investigated over the last thirty‐five years, initially as prospective antibiotics in their own right and inhibitors of the ‐lactamase enzymes that impart resistance to ‐lactams, more recently as inhibitors of other serine proteases and mechanistic probes of ‐lactam biosynthesis. Cyclobutanone analogues of the penam ring system are the first reversible inhibitors to demonstrate moderate activity against all classes of ‐lactamase, while other compounds from this family inhibit Streptomyces R61 DD‐carboxypeptidase/transpeptidase, human neutrophil elastase (HNE) and porcine pancreatic elastase (PPE). But has their potential as enzyme inhibitors been fully exploited? Challenges in synthesising diversely functionalised derivatives mean only a limited number and structural diversity of cyclobutanone ‐lactam analogues have been made and evaluated to date. This review surveys the different synthetic approaches that have been taken to these compounds, investigations to evaluate their biological activity, and prospects for future developments in this area. Key words ‐lactam, ‐lactamase inhibitor, cyclobutanone, antibiotics, antimicrobial resistance, penicillin, cephalosporin, carbapenam 1 Introduction The emergence of antimicrobial resistance to antibiotics as a major global problem is well documented in both the scientific literature and the popular press.[1‐7] ‐Lactamases and ‐lactam antibiotics are key players in this emerging epidemic: ‐lactams are amongst the most widely used and best understood classes of antibiotics, ‐lactamases pose an ever‐increasing threat to their continued efficacy as they hydrolyse ‐lactams before they can exert an antibiotic effect.[8‐10] ‐Lactam antibiotics have been characterised in great detail over the almost 90 years since Fleming’s serendipitous discovery of Penicillium‐mediated antibiosis:[11] their biosynthesis and modes of action, mechanisms of resistance to ‐lactams, and methods for overcoming that resistance have all been investigated in depth.[12‐15] Myriad structural variants have been conceived and tested for activity as antibiotics and as ‐lactamase inhibitors to combat resistance. The gamut of ‐lactam ring systems characterised ranges from the penam 1 first discovered to oxycyclic clavam 2, unsaturated penem 3, oxapenem 4 and carbapenem 5 ring systems, the ring‐ expanded cephems 6, oxacephems 7 and carbacephems 8 (also unsaturated) and the monocyclic monobactams 9 and nocardicins 10 (Scheme 1). These subfamilies of compounds all retain the ‐ lactam ring and vary elsewhere in their structure. The chemistry and biochemistry of these systems has been reviewed comprehensively elsewhere.[15‐20] The focus of this review is ‐lactam analogues that are not themselves ‐lactams: cyclobutanone derivatives designed to mimic the structure and activity of ‐lactams without actually incorporating the signature four‐membered cyclic amide. Cyclobutanone is isosteric with the ‐lactam ring, with the quintessential ‐lactam nitrogen replaced by a carbon (Scheme 1). Since the ‐lactam nitrogen is effectively pyramidal by virtue of ring fusion in the penam and cephem ring systems, Gordon Lowe and others hypothesised that 2 replacing this nitrogen with an sp3 hybridised carbon should “retain stereochemical compatibility with the active site of the transpeptidases and D,D‐carboxypeptidases involved in bacterial cell wall biosynthesis.”[21] Thus cyclobutanone analogues of ‐lactams have been explored since the early 1980s, as potential antibiotics in their own right,[21‐24] as ‐lactamase and serine protease inhibitors,[21, 24‐28] and as mechanistic probes.[29‐31] Scheme 1: General structures for the major subfamilies of ‐lactams studied to date. The penam 1, clavam 2, carbapenem 5, cephem 6, monobactam 9 and nocardicin 10 ring systems are naturally occurring ‐lactams, while penems 3, oxapenems 4, oxacephems 7 and carbacephems 8 are synthetic ‐lactam subfamilies. The inset shows a generalized cyclobutanone analogue 11 of the penam 1: the tertiary nitrogen of the ‐lactam is replaced by a carbon (CH). R, R’ and R” = various. It has been proposed that cyclobutanones – such as the generalised penam analogue 11 – have the potential to inhibit serine ‐lactamases via formation of an enzyme bound hemiketal with the active site serine (Scheme 2a), and metallo ‐lactamases via oxygen coordination to the metal ions (Scheme 2b).[21, 27, 28] Further, it has been postulated that cyclobutanone analogues may inhibit serine proteases more generally, by hemiketal formation akin to that shown for the serine ‐ lactamase in Scheme 2a.[22, 25, 32] 3 Scheme 2: Proposed activity of cyclobutanones as broad‐spectrum ‐lactamase inhibitors, shown for a generalized cyclobutanone‐penam 11: inhibition of a. serine ‐lactamases via hemiketal formation, and b. metallo‐‐lactamases via oxygen coordination to zinc. Adapted with permission from Johnson et al. J. Org. Chem. 2008, 73, 6970‐6982. Copyright 2008 American Chemical Society. R = various. Cyclobutanone analogues of the penam, clavam and carbapenam ring systems, and various monocyclic analogues, have all been synthesised and evaluated as inhibitors of transpeptidases, ‐ lactamases and other proteases. Early efforts in this field were reviewed by Jungheim and Ternansky in the early 1990s, part of their chapter on Non‐‐lactam mimics of ‐lactam antibiotics[33] in the comprehensive text The Chemistry of ‐Lactams.[34] Much has happened since, and the aim of this review is to bring together almost forty years of collective endeavour, surveying and evaluating the synthetic approaches developed to make these challenging targets, the application of these compounds as enzyme inhibitors and mechanistic probes, and possible avenues for further investigation. Synthesis A variety of synthetic routes to cyclobutanone‐‐lactam analogues have been developed. All routes to bicyclic derivatives exploit [2+2] cycloaddition reactions to construct the four‐membered cyclic ketone.[22, 35] Early Routes Gordon et al., reported the first synthesis of cyclobutanone analogues of penam and penem rings systems in 1981;[22] these were carbapenam and carbapenem analogues (Scheme 3), conceived as DD‐carboxypeptidase and transpeptidase enzyme inhibitors. 4Carboxybicyclo[3.2.0]heptane‐6‐ 4 one 12 was conceived as a simplified isosteric model of penicillanic acid 13, with both the ‐lactam N and thiazolidine S replaced by carbons, while 4carboxybicyclo[3.2.0]hept‐2‐ene‐6‐one 14 is the cyclobutanone isostere of the unfunctionalised carbapenem 15. Scheme 3: First generation cyclobutanone analogues 12 and 14, conceived as mimics of penicillanic acid 13 and the simple carbapenem 15 respectively.[22] Both 12 and 14 were synthesized in racemic form from 6,6‐bis‐methylmercaptofulvene 16 and dichloroketene (generated in situ from dichloroacetyl chloride and an amine base) via a [2+2] cycloaddition reaction to form cycloadduct 17 (Scheme 4).[35] Cleavage of the mercaptofulvene group in 17 using formic acid and two equivalents of mercuric chloride produced both the carboxylic acid 18 and thioester 19 in a 1:4 ratio, and 16% combined yield over three steps from cyclopentadiene, precursor to fulvene 16. Carboxylic acid 18 and thioester 19 were each isolated as a single diastereomer (Scheme 4), their regiochemistry and relative stereochemistry confirmed by comparison of 1H NMR data with literature data for related compounds, and X‐ray crystallographic analysis of 18. From the carboxylic acid 18, reductive dechlorination using zinc and acetic acid followed by catalytic hydrogenation afforded carbapenam‐cyclobutanone 12. From thioester 19, hydrolysis using a mixture of concentrated hydrochloric acid and glacial acetic acid, then the zinc/ acetic acid dechlorination, gave carbapenem analogue 14. 5 Scheme 4: Synthesis of first generation cyclobutanone analogues 12 and 14 (racemic). Reagents and conditions: (i) Cl2CHCOCl, Et3N, Et2O, ‐10 °C; (ii) HCOOH, HgCl2, reflux, 15 min, 16% over 3 steps, of a ~1:4 mixture of 18:19; (iii) Zn/HOAc, 70 °C, 45 min, 66%; (iv) H2, Pd/C, EtOAc, 82%; (v) HCl/HOAc, 27%; (vi) Zn, HOAc, 70 °C.[35] Meth‐Cohn et al. extended this methodology to make the gem‐dimethyl analogue 20 (Scheme 5).[23] Their approach used the [2+2] cycloaddition of dimethylketene to cyclobutadiene 21 to generate the fused bicyclic system 22, but then deployed a cyanide‐mediated rearrangement and a series of reductive functional group interconversions (FGIs) such that the carbon atoms of the ketene ultimately reside in the five‐membered ring, and not the cyclobutanone. Thus bicyclic
Details
-
File Typepdf
-
Upload Time-
-
Content LanguagesEnglish
-
Upload UserAnonymous/Not logged-in
-
File Pages30 Page
-
File Size-