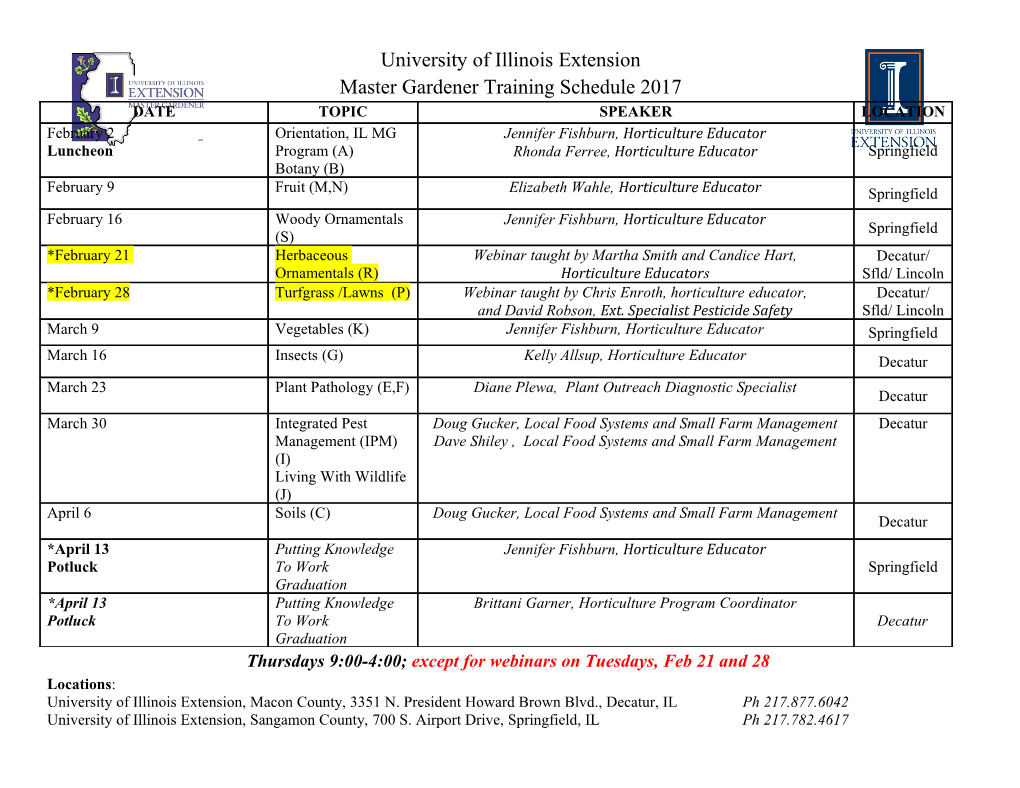
Tight Control of Nitrate Acquisition in a Plant Species that Evolved in an Extremely Phosphorus-Impoverished Environment M. Asaduzzaman Prodhan1*, Ricarda Jost1, Mutsumi Watanabe2, Rainer Hoefgen2, Hans Lambers1 & Patrick M. Finnegan1 1School of Plant Biology, The University of Western Australia, 35 Stirling Highway, Perth, WA 6009, Australia; 2Max Planck Institute for Molecular Plant Physiology, Am Mühlenberg 1, Potsdam-Golm D- 14476, Germany * Author for correspondence: M. Asaduzzaman Prodhan Tel: +61 430079374 E-mail: [email protected]; [email protected]; [email protected] 1 Abstract Hakea prostrata (Proteaceae) has evolved in an extremely phosphorus- (P) limited environment. This species exhibits an exceptionally low ribosomal RNA (rRNA) and low protein and nitrogen (N) concentration in its leaves. Little is known about the N requirement of this species, and its link to P metabolism, despite this being the key to understanding how it functions with a minimal P budget. Hakea prostrata plants were grown with various N supplies. Metabolite and elemental analyses were performed to determine its N requirement. Hakea prostrata maintained its organ N content and concentration at a set-point, independent of a 25-fold difference nitrate supplies. This is in sharp contrast to plants that are typically studied, which take up and store excess nitrate. Plants grown without nitrate had lower leaf chlorophyll and carotenoid concentrations, indicating N deficiency. However, H. prostrata plants at low or high nitrate availability had the same photosynthetic pigment levels, and hence were not physiologically compromised by the treatments. The tight control of nitrate acquisition in H. prostrata retains protein at a very low level, which results in a low demand for rRNA and P. We surmise that the constrained nitrate acquisition is an adaptation to severely P-impoverished soils. Key words: Hakea prostrata, metabolite profiling, nitrogen uptake, phosphorus-use efficiency, plant nutrition, Proteaceae. 2 Introduction Hakea prostrata (Proteaceae) is native to south-western Australia, a region that has some of the most severely P-impoverished soils on earth (Lambers et al. 2015, Lambers et al. 2011). This species has evolved in an environment with soils that contain as little as nine to 25 mg total P kg-1 dry soil (Lambers et al. 2012b), which is only five percent of that generally found in unfertilised crop or pasture soils (550-770 mg total P kg-1 dry soil) (Hedley et al. 1982). Adaptions that allow this species to survive on these extremely P-impoverished soils include its ability to form cluster roots, specialised dense clusters of lateral roots that exude large amounts of organic anions (carboxylates) into a small volume of soil to release P from insoluble P-complexes (Lambers et al. 2010). In addition, this species maximises its internal P-use efficiency by i) efficiently and proficiently remobilising P from senescing leaves for re- use (Lambers et al. 2012a), ii) extensively replacing P-containing lipids during leaf development with lipids that do not contain P without compromising photosynthesis (Lambers et al. 2012b), and iii) functioning at very low levels of rRNA (Sulpice et al. 2014), the largest pool of organic P in leaf cells (Veneklaas et al. 2012). The low abundance of rRNA implies a slow rate of protein synthesis (Elser et al. 1996, Matzek & Vitousek 2009), consistent with the very low concentrations of leaf protein and N in H. prostrata (Sulpice et al. 2014). Here we hypothesised that the low abundance of rRNA and total leaf N in H. prostrata is linked to a tight control of N acquisition and assimilation. 3 Materials and Methods Plant material and growth conditions Hakea prostrata R.Br. plants were grown in a temperature-controlled glasshouse at the University of Western Australia during May to August 2014. Six-month-old soil-grown plants were transferred to a hydroponics system with a constant root temperature of 18 to 20 °C. Roots were first washed and disinfected with 0.01% (v/v) sodium hypochlorite for eight min. Plants were transferred into five litre (L) black plastic pots, one plant per pot, containing four L nutrient solution (100 µM Ca(NO3)2, 100 µM K2SO4, 2.5 µM KH2PO4, 27 µM MgSO4, 0.1 µM MnCl2, 0.1 µM ZnSO4, 0.025 µM CuSO4, 1.25 µM H3BO3, 0.0075 µM CoCl2, 0.025 µM (NH4)6Mo7O24, 5 µM Fe-EDTA, 10 µM KCl, 33.3 µM Na2O3Si; pH 5.8). The nutrient solution was changed twice a week for six weeks to allow plants to acclimate to the hydroponics system. Average minimum and maximum temperatures were 14 °C at night and 25 °C during the day, and the average maximum light intensity (70% transmission) between 12:00 h and 14:00 h was 680 µmol m-2 s-1, with sunrise at 07:15 h and sunset at 17:30 h. Relative humidity averaged 32% during the day and 62% at night. Nutrient treatments and plant harvest After the initial acclimation period, the phosphate and nitrate supplies were varied independently. Each treatment was applied to four randomly-assigned replicate plants. A further four plants were harvested immediately before starting the treatments to determine - their initial metabolic status. Treatments were binary combinations of doses of H2PO4 to a - final concentration of 10 µM or 20 µM, and NO3 to a final concentration of 0, 200 µM or 5000 µM applied twice per week when the entire nutrient solution was replaced. Treatments were applied for five weeks. Plants were visually assessed for nutrient deficiency or toxicity 4 symptoms at each change of solution. At harvest, five to six of the most recently fully expanded mature leaves on the main stem were bulked together, weighed, immediately frozen in liquid nitrogen and stored at -80 °C to be analysed later. The remaining parts of the plant were weighed immediately to determine fresh weights, oven dried at 65 °C for a week, and weighed again to determine dry weights. Snap-frozen mature leaves were used to determine nitrate, phosphate, individual amino acids, total soluble protein and chlorophyll concentrations. Total N and P concentrations were determined in oven dried mature leaves, stems and roots. Total N and P content in leaves, stems and roots were calculated from leaves, stems and roots N and P concentrations and their biomass, respectively. Determination of total N and P Approximately 0.2 g ground oven-dried plant material was digested with 2 ml of H2SO4 for 30 s and 0.5 ml of H2O2 for 10 to 15 min at 320 °C. Samples were allowed to cool for up to 15 min before placing them again at 320 °C for 45 min to ensure complete oxidation. Following evaporation of H2O2, the sample volume was made up to 75 ml with Milli-Q water and used to determine the concentration of total N and P(Technicon 1977, Wolf 1982). Determination of total soluble protein An aliquot of 20 mg cryogenically ground plant material was extracted in pre-cooled tubes three times for 30 min each at 120 °C. The first extraction was with 250 µl 80% (v/v) ethanol (buffered with 10 mM 2-(N-morpholino) ethanesulfonic acid, pH 5.9). The second extraction was with 150 µl of the same solution. The final extraction was with 250 µl 50% (v/v) ethanol buffered as above. At the end of each step, the extract was centrifuged for 10 minutes at 5200 x g. The supernatant from the extraction was discarded and the pellet resuspended in 400 µl of 0.1 M NaOH, mixed vigorously and heated at 120 °C for 30 minutes. After cooling to 25 °C, the suspension was centrifuged at 10,620 x g for 10 min. An aliquot of the supernatant 5 was used to spectrophotometrically determine the total soluble protein using the Bradford method(Bradford 1976). Determination of pigments A 25 mg aliquot of cryogenically ground leaf tissue was homogenised in 2 ml methanol. The mixture was incubated at 4 °C in the dark for 30 min, before clearing by centrifugation for 5 min at 21,000 x g. The absorbance of the supernatant at 470, 653 and 666 nm was determined. Chlorophyll a and b and total carotenoid concentrations were calculated using their specific absorption coefficients in methanol and published equations(Lichtenthaler & Wellburn 1983, Warren 2008). Sample preparation for determination of amino acids and ions Snap-frozen plant material was ground cryogenically (Cryorobot, Labman, North Yorkshire, UK), and aliquoted for multiple analyses. Fifty mg of ground material was extracted with 360 µl pre-cooled (-20°C) extraction mixture (300 µl methanol, 30 µl two mg ml-1 nonadecanoic -1 13 acid methylester in CHCl3, 30 µl 0.2 mg ml C6-sorbitol in methanol) for 15 min at 70°C with 550 rpm shaking. Pressure was released at one min intervals by quickly opening and closing the cap of the tube. After cooling the solution to 25°C, 200 µl CHCl3 was added. The solution was mixed vigorously and incubated for five min at 37°C with 600 rpm shaking. The polar fraction was prepared by liquid partitioning into 400 μL of ULC/MS (ultra liquid chromatography/mass spectrometry) grade water followed by vigorous mixing. The polar and lipid phases were separated by centrifuging for five min at 21,000 x g at 25°C. Four 100 µl aliquots of the supernatant polar phase were dried by rotary evaporation under vacuum. 6 Determination of individual amino acids Vacuum-dried 100 µl aliquots of samples were dissolved in 45 µl five mM sodium phosphate buffer (pH 6.2) by vigorous mixing. Solutions were centrifuged at 21,000 x g for 15 min at 4°C.
Details
-
File Typepdf
-
Upload Time-
-
Content LanguagesEnglish
-
Upload UserAnonymous/Not logged-in
-
File Pages97 Page
-
File Size-