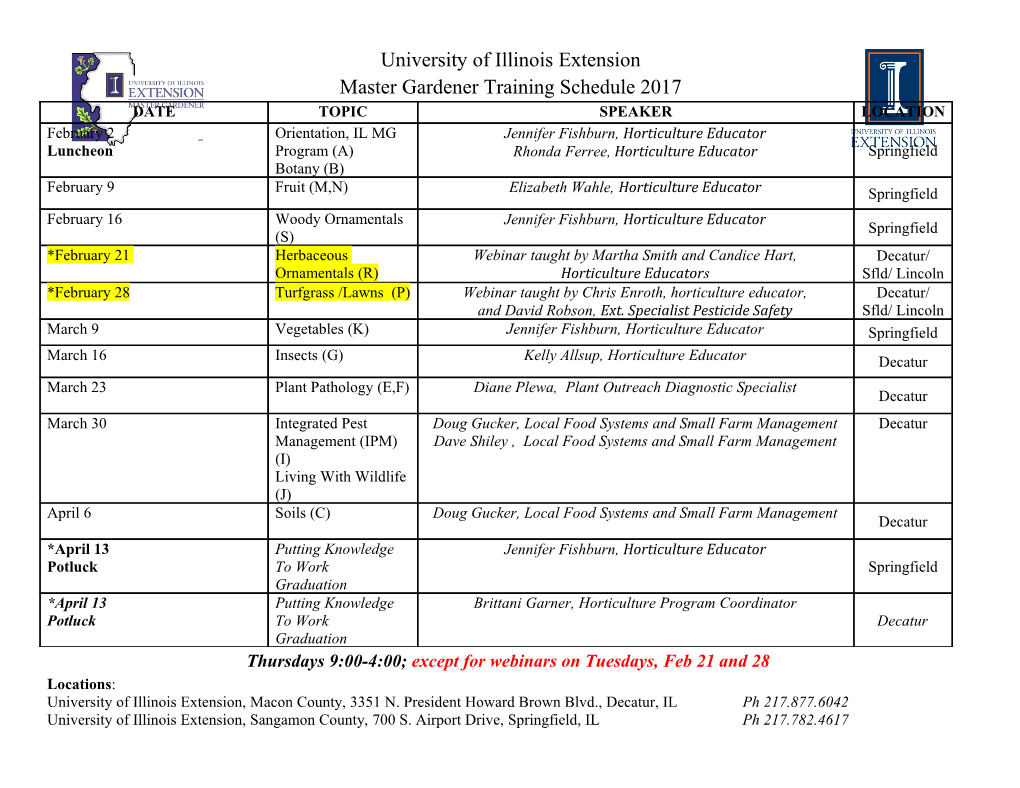
International Journal of Molecular Sciences Review In Vitro Innovation of Tendon Tissue Engineering Strategies 1, , 2, 1 Maria Rita Citeroni * y, Maria Camilla Ciardulli y, Valentina Russo , Giovanna Della Porta 2,3 , Annunziata Mauro 1 , Mohammad El Khatib 1 , Miriam Di Mattia 1 , Devis Galesso 4 , Carlo Barbera 4, Nicholas R. Forsyth 5 , Nicola Maffulli 2,6,7,8 and Barbara Barboni 1 1 Unit of Basic and Applied Biosciences, Faculty of Bioscience and Agro-Food and Environmental Technology, University of Teramo, 64100 Teramo, Italy; [email protected] (V.R.); [email protected] (A.M.); [email protected] (M.E.K.); [email protected] (M.D.M.); [email protected] (B.B.) 2 Department of Medicine, Surgery and Dentistry, University of Salerno, Via S. Allende, 84081 Baronissi (SA), Italy; [email protected] (M.C.C.); [email protected] (G.D.P.); n.maff[email protected] (N.M.) 3 Interdepartment Centre BIONAM, Università di Salerno, via Giovanni Paolo I, 84084 Fisciano (SA), Italy 4 Fidia Farmaceutici S.p.A., via Ponte della Fabbrica 3/A, 35031 Abano Terme (PD), Italy; DGalesso@fidiapharma.it (D.G.); CBarbera@fidiapharma.it (C.B.) 5 Guy Hilton Research Centre, School of Pharmacy and Bioengineering, Keele University, Thornburrow Drive, Stoke on Trent ST4 7QB, UK; [email protected] 6 Department of Musculoskeletal Disorders, Faculty of Medicine and Surgery, University of Salerno, Via San Leonardo 1, 84131 Salerno, Italy 7 Centre for Sports and Exercise Medicine, Barts and The London School of Medicine and Dentistry, Mile End Hospital, Queen Mary University of London, 275 Bancroft Road, London E1 4DG, UK 8 School of Pharmacy and Bioengineering, Keele University School of Medicine, Thornburrow Drive, Stoke on Trent ST5 5BG, UK * Correspondence: [email protected]; Tel.: +39-(347)-940-0970 These authors contributed equally to this review. y Received: 19 August 2020; Accepted: 7 September 2020; Published: 14 September 2020 Abstract: Tendinopathy is the term used to refer to tendon disorders. Spontaneous adult tendon healing results in scar tissue formation and fibrosis with suboptimal biomechanical properties, often resulting in poor and painful mobility. The biomechanical properties of the tissue are negatively affected. Adult tendons have a limited natural healing capacity, and often respond poorly to current treatments that frequently are focused on exercise, drug delivery, and surgical procedures. Therefore, it is of great importance to identify key molecular and cellular processes involved in the progression of tendinopathies to develop effective therapeutic strategies and drive the tissue toward regeneration. To treat tendon diseases and support tendon regeneration, cell-based therapy as well as tissue engineering approaches are considered options, though none can yet be considered conclusive in their reproduction of a safe and successful long-term solution for full microarchitecture and biomechanical tissue recovery. In vitro differentiation techniques are not yet fully validated. This review aims to compare different available tendon in vitro differentiation strategies to clarify the state of art regarding the differentiation process. Keywords: in vitro; tendon differentiation; stem cells 1. Introduction Tendons connect muscles to bones and allow movements. Lesions and inflammation can occur in tendons because of mechanical stress, aging, genetic predisposition, or lesions and inflammation [1]. Int. J. Mol. Sci. 2020, 21, 6726; doi:10.3390/ijms21186726 www.mdpi.com/journal/ijms Int. J. Mol. Sci. 2020, 21, 6726 2 of 78 Tendinopathy is term used with refering to tendon disorders, and it is a generic descriptive term for the clinical condition in and around tendons arising from overuse [1]. The major conditions affecting tendons are tendinitis and tendinosis; the first is characterized by inflammation and pain, while the second is probably caused by tendinous degeneration [2]. In tendinopathy, the homeostasis of the tissue may be deeply affected resulting in permanent changes of the native tendon structures and mechanics [3]. Spontaneous adult tendon healing results in scar tissue formation and fibrosis, and it is accompanied by alterations in the biomechanical properties of the tissue. Adult tendons have a limited natural healing capacity and often respond poorly to current treatments focused on exercise, drug delivery, and surgical procedures [3]. The incapacity of complete healing derives from the nature of tendon with its poor cellularity, limited vascularization, and low metabolism [3,4]. The cellular component of the tendon is very low, and with age, it tends to diminish and change in morphology, with loss of stemness markers [5–7]. As the tendon is a mechanosensitive tissue and extracellular matrix (ECM) remodeling is influenced by mechanical stimulation [8,9], prolonged rehabilitation is considered a valid alternative to surgery that offers great support and is more efficient than pharmacological therapy. Regeneration, studied in fetal healing, is characterized by restoration of the native structural and functional properties of the tissue, without scar formation [10]. Therefore, it is of great importance to identify key molecular and cellular processes involved in the progression of tendinopathies and in tendon response to them to develop effective therapeutic strategies and drive the tissue toward regeneration. Unfortunately, the understanding of tendon biology and healing is incomplete, and the development of innovative treatment modalities is still lagging behind increasing demands [1]. To treat tendon diseases and support tendon regeneration, cell-based therapy, as well as tissue engineering approaches, are now considered as potential approaches to reproduce a safe and successful long-term solution for the full microarchitecture and biomechanical tissue recovery. The first step prior to any stem cell-based procedure or tissue-engineered approach to improve tendon healing and regeneration in vivo is the in vitro model. In vitro techniques are fundamental to: Identify and/or compare the tenogenic plasticity of different stem/progenitor cell sources, • Define and drive cell mechanism and environmental conditions leading tenogenesis, • Control step-wise signaling molecules and pathways, • Direct stem cell pre-commitment before transplantation (reducing tumorigenic risks with • embryonic stem cells (ESCs), unwilling differentiation path of mesenchymal stem cells (MSCs) or to increase tissue integration), Study the tenogenic properties of stem cells, • Test teno-inductive properties of new scaffolds, • Validate biomechanical teno-inductive stimuli. • At this current time, in vitro differentiation techniques are not yet validated. This review aims to compare available in vitro tendon differentiation strategies to clarify the state of art with respect to a differentiation process that remains an open biological challenge for researchers, bioengineers, and clinicians. We will begin with tendon structure, functions, and biology before describing different sources of stem cells, and finally proceeding with collating the most used tendon-inductive techniques (hypoxia, physical stimuli, biomaterials, growth factor, and co-culture) (Figure1). Int. J. Mol. Sci. 2020, 21, 6726 3 of 78 Int. J. Mol. Sci. 2020, 21, x 3 of 78 Figure 1. In vitro strategies for tendon tissue engineering. Tendon tissue engineering refers to aFigure multidisciplinary 1. In vitro strategies field that for aims tendon at the tissue inducement engineer ofing. tissue Tendon repair tissue or regeneration. engineering Therefore,refers to a it involvesmultidisciplinary the combination field that of aims several at keythe factors,inducement such of as tissue cells, scarepairffolds, or biochemicalregeneration. and Therefore, mechanical it inputsinvolves to the produce combination a functional of several tendon-like key factors, construct. such as cells, Abbreviations. scaffolds, biochemical PGA: polyglycolic and mechanical acids; PLA:inputs polylactic to produce acids, a functional PCL: polycaprolactones; tendon-like construct. PLGA: Abbreviations. poly(lactic-co-glycolic) PGA: polyglycolic acids; acids; PLCL: PLA: poly (lactil-co-captolactone)polylactic acids, PCL: polycaprolactones; acids; ESCs: embryonic PLGA: stem poly(lactic-co-glycolic) cells; iPSCs: induced acids; pluripotent PLCL: stempoly cells;(lactil-co- AECs: amnioticcaptolactone) epithelial acids; stem ESCs: cells; embryonic AMCs: amniotic stem cells; mesenchymal iPSCs: induced stem cells;pluripotent AFCs: amnioticstem cells; fluid AECs: stem cells;amniotic UB-MSCs: epithelial umbilical stem cells; cord AMCs: mesenchymal amniotic stemmesenchymal cells; BMSCs: stem bonecells; marrowAFCs: amniotic mesenchymal fluid stem stem cells;cells; ADSCs:UB-MSCs: adipose umbilical derived cord mesenchymal mesenchymal stem stem cells; cells; TPSCs: BMSCs: tendon bone progenitorsmarrow mesenchymal stem cells; TGFstemβ : transformingcells; ADSCs: growth adipose factor derived beta; mesenchymal BMPs: bone stem morphogenetic cells; TPSCs: proteins; tendon CTGF: progenitors connective stem tissuecells; TGF growthβ: factor;transforming FGFs: fibroblasticgrowth factor growth beta; factors; BMPs: IGF-1:bone morphogenetic VEGF: vascular pr endothelialoteins; CTGF: growth connective factor; PDGFs:tissue platelet-derivedgrowth factor; FGFs: growth fibroblastic factor. growth factors; IGF-1: VEGF: vascular endothelial growth factor; 1.1. TendonPDGFs: Structure platelet-derived growth factor. 1.1. TendonTendons Structure are fibro-elastic structures that connect
Details
-
File Typepdf
-
Upload Time-
-
Content LanguagesEnglish
-
Upload UserAnonymous/Not logged-in
-
File Pages78 Page
-
File Size-