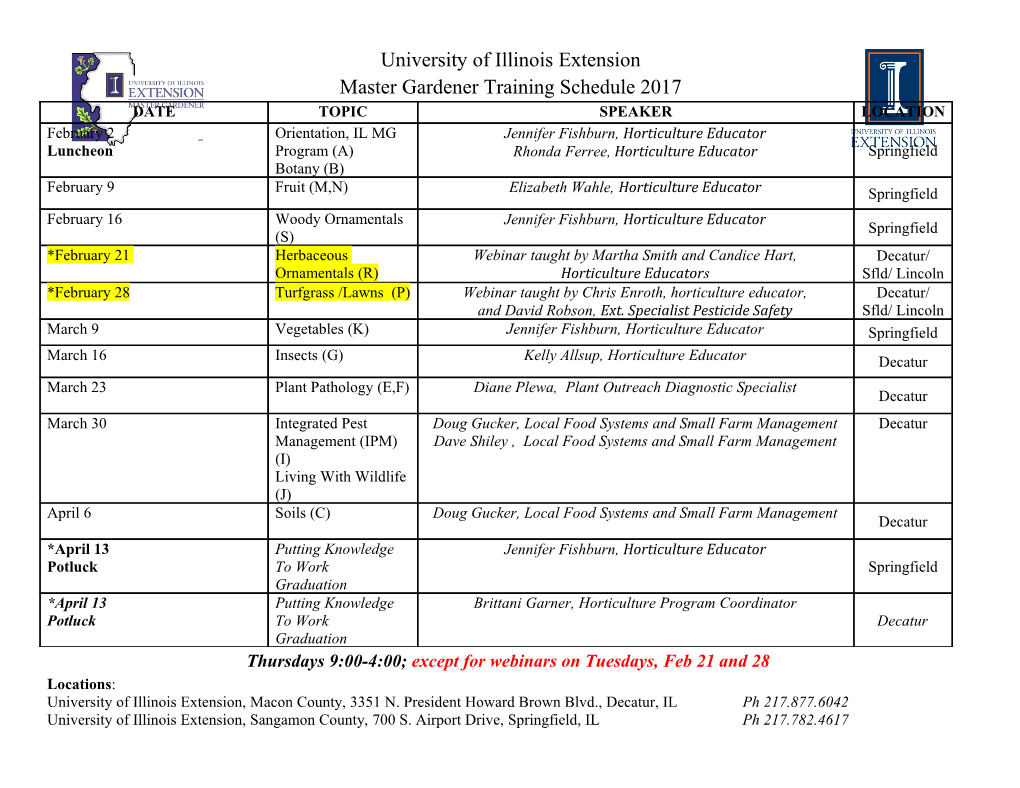
Astronomy 218 Lives of Other stars russell-Vogt Theorem Henry Norris Russell and Heinrich Vogt were the first to note the influence of a star’s mass. “A star’s properties are determined primarily by its mass.” The pre-main sequence behavior is one example. The stellar lifetime is also determined by the star’s mass, τ ∝ M−3. The mass has an even larger impact on the star’s destiny. Leaving the Main Sequence After a main sequence life that varies from 1010 years to 107 years, differences appear from the point of hydrogen exhaustion in the stellar core. Stars more massive than the Sun follow different paths. A 4 M☉ star takes a long looping path before reaching the Hayashi line and climbing in radius. A 10 M☉ star loops smoothly back and forth between blue supergiant and red supergiant. Structural Differences While the post-main sequence events for stars more massive than the Sun follow the same sequence as those in lower- mass stars, the structure of more massive stars respond differently. Stars with M ≳ 1.5 M☉ have convective cores due to the CNO cycle, but radiative envelopes. Envelope opacity changes gradually until the Hayashi limit, where cool temperatures raise the opacity dramatically, is reached. The details of the loops depends on the interplay of opacity, nuclear energy generation, metallicity, and theory of convection, thus models of the loops remain uncertain. Stars with M ≳ 10 M☉ never reach the Hayashi limit. Helium White Dwarf Achieving the temperatures (> 108K) and densities (> 107 kg m−3) needed for helium burning requires the helium core to exceed 0.45 M☉, preventing stars of ≲ 0.5 M☉ from ever burning helium. Thus they would end their lives as helium white dwarves. However, with a lifetime more than 8 times the Sun’s, it is unlikely that any 0.5 M☉ star has yet exhausted its hydrogen core. Nonetheless white dwarves with masses less than 0.3 M☉ have been found in globular clusters. Helium Flash? There is a general trend among stars of higher mass to have lower central densities for the same temperature. This difference is responsible for the mass-luminosity relation and has other effects. 8 The range in density at 10 K allows stars with M > 2.5 M☉ to begin helium burning under non-degenerate conditions, thus they do not experience a helium flash. Carbon Flash? A star of mass > 8 M☉ can ignite carbon burning in its core, leading to a different fate than the Sun. Carbon fusion can have a number of results 12C + 12C → 24Mg + γ unlikely, EM reaction → 20Ne + α winner! → 23Ne + p captures p → 20Ne + α → 23Mg + n decays to 23Ne → 16O + α + α unlikely, 3 body For stars of ~8-10 M☉, this ignition occurs under degenerate circumstances, likely producing a carbon flash. This represents the final nuclear state of the star, leaving a white dwarf composed primarily of oxygen and neon with small amounts of magnesium and sodium, an ONe (or ONeMg) white dwarf. Iron Core A star that manages to ignite carbon under non-degenerate circumstances can continue to fuse elements up to iron. Fusion reaction after iron are energetically unfavorable, which will have dire consequences. For a 20 M☉ star carbon burning (and subsequent burning stages) occur over less than a thousand years. These stages do not affect the stellar surface and are therefore invisible. Mass Loss from Giants All stars lose mass via some form of stellar wind, with metal- rich stars generally losing mass more rapidly. Sun-like stars have small rates of mass loss, except during their post-main sequence life as red giant and AGB stars −3 −6 −1 (10 -10 M☉ yr ). The most massive stars have strong winds throughout their lifetimes. O- and B-type stars can lose a tenth of their total −5 −6 −1 mass via stellar winds in a million years (10 -10 M☉ yr ) on the main sequence. These winds remove sufficient mass to alter stellar evolution. Wolf-Rayet stars are helium stars thought to occur when winds remove the hydrogen envelopes of massive stars (M >20 M☉) . Visualizing Winds Aside from their affect on their parent star’s evolution, these stellar winds affect the interstellar environment, hollowing out cavities in the ISM around giant stars. Crescent Nebula Non-Destructive Pulsations Not all stellar pulsations are as violent or destructive as those seen in AGB star. Many giants exhibit excursions in radius. These variations are detectable as they cause the luminosity to vary in a periodic fashion. Such stars are called intrinsic variables. Each class of intrinsic variable has a physical instability which drives the pulsations. Two observed types of intrinsic variables, RR Lyrae stars and Cepheids share a common instability. For these stars in the instability is driven by the increased opacity of ionized helium. The transition from He+ → He++ frees an additional electron, increasing the Thomson opacity. Periodic Brightness RR Lyrae stars have similar light curves with periods from 8-24 hours. Cepheids, named for δ Cephei, are brighter stars which exhibit longer periods, ranging from about 1 to 100 days. For example, the brightness of δ Cephei exhibits a change of Δmv ~ 1 over a period of 5.4 days. A 15% radial expansion of δ Cephei is detectable through changes in mv, Teff (6600K → 5500K) and its radial velocity (varies from −35 km s−1 to +5 km s−1). Instability Strip The population of RR Lyrae and Cepheid variable stars marks a strip in the HR diagram where this He+ → He++ instability occurs. RR Lyrae variables are horizontal branch stars between 0.5-0.7 M☉, generally more metal-poor than the Sun. Cepheid variables are more massive (3-18 M☉) supergiant stars with high metallicity. Polaris is the nearest example of a Cepheid. Measuring Distances The usefulness of these stars comes from their period– luminosity relation. Studies of the Small and Large Magellanic Clouds by Henrietta Swan Leavitt in 1912 revealed a correlation between apparent magnitude and log P. The modern form of the relation is M̅ V = −2.76 log (P/10 days) − 4.16 Measuring a period yields the luminosity. Combining with the apparent brightness reveals the distance. Acoustic Oscillations Stellar pulsations results from pressure waves that travel between the core and surface. Sound waves whose wavelength are integral multiples of the radius become standing waves or acoustic oscillations. The oscillation period is determined by the sound crossing time, Using hydrostatic equilibrium, we can approximate which yields Thus the period is much longer for supergiants. Eddington Valve The Eddington Valve or κ Mechanism was first proposed in 1917: “If a layer became more opaque upon compression it could “dam up” the energy flowing to the surface and push the surface layers upward”. Physically, most opacities do not function in this fashion, but ionization can Compression ⇒ more ionization ⇒ increases opacity Expansion ⇒ recombination ⇒ decreases opacity The Eddington valve is thought to underly most regular stellar pulsations. S. A. Zhevakin in 1953 first ascribed the Cepheid Instability strip to the transition from He+ → He++ . Testing Stellar Evolution Since most stages of stellar evolution last much longer than the total extent of human civilization, we are unable to test our theory of stellar evolution against the life of an individual star. We can test our theory against clusters of stars. 47 Tucanae Failing the Test? We have already seen that observations of globular clusters reveal helium white 47 Tucanae dwarves which should take longer than the age of the Universe to form. Cluster H-R diagrams often reveal stars remaining on the main sequence to the left of the red giant runoff. These Blue Stragglers challenge the Russell-Vogt theorem. Is our theory of stellar evolution flawed? Binary-Star Evolution The fact that both of the helium white dwarf and blue straggler problems are most noticeable in globular clusters, with their high density of stars, provides a clue to the cause. Binary stars can evolve differently from solitary stars. If the stars in a binary-star system are relatively widely separated, their evolution proceeds much as it would have if they were solitary stars. If they are closer, it is possible for material to transfer from one star to another, changing the mass of both the donor and recipient stars, leading to unusual evolutionary paths. Close requires orbital separations ~ stellar radius, which means that interactions are more common in the giant phase. Binary-Star Vocabulary Each star is surrounded by its Roche Lobe. Within its Roche lobe, each star’s gravity is dominant. The Roche Lobes of binary companions intersect at the L1 Lagrangian point. Here the gravitational forces are equal. Binary systems are categorized by how close the stars are. In a detached binary, each star is separate. In a semidetached binary, one star fills its Roche Lobe. In a contact binary, both Roche Lobes are (over) filled. Algol Algol is a nearby (29 pc) eclipsing binary system consisting of a blue main sequence star (B8V) and a red sub-giant (K2IV). Surprisingly, the masses of the stars are reversed from our expectation: MA = 3.6 M☉ and MB = 0.8 M☉, making the less massive star more evolved. One possibility is that the stars have different ages, requiring the binary to have formed by capture. This is unlikely for field stars, so we believe Algol formed as a detached binary and evolved together. Mass Transfer As the initially more massive blue-giant star entered its red- giant phase, it expanded to become a semi-detached binary. At this point mass transfer occurred, growing the mass of the second star.
Details
-
File Typepdf
-
Upload Time-
-
Content LanguagesEnglish
-
Upload UserAnonymous/Not logged-in
-
File Pages24 Page
-
File Size-