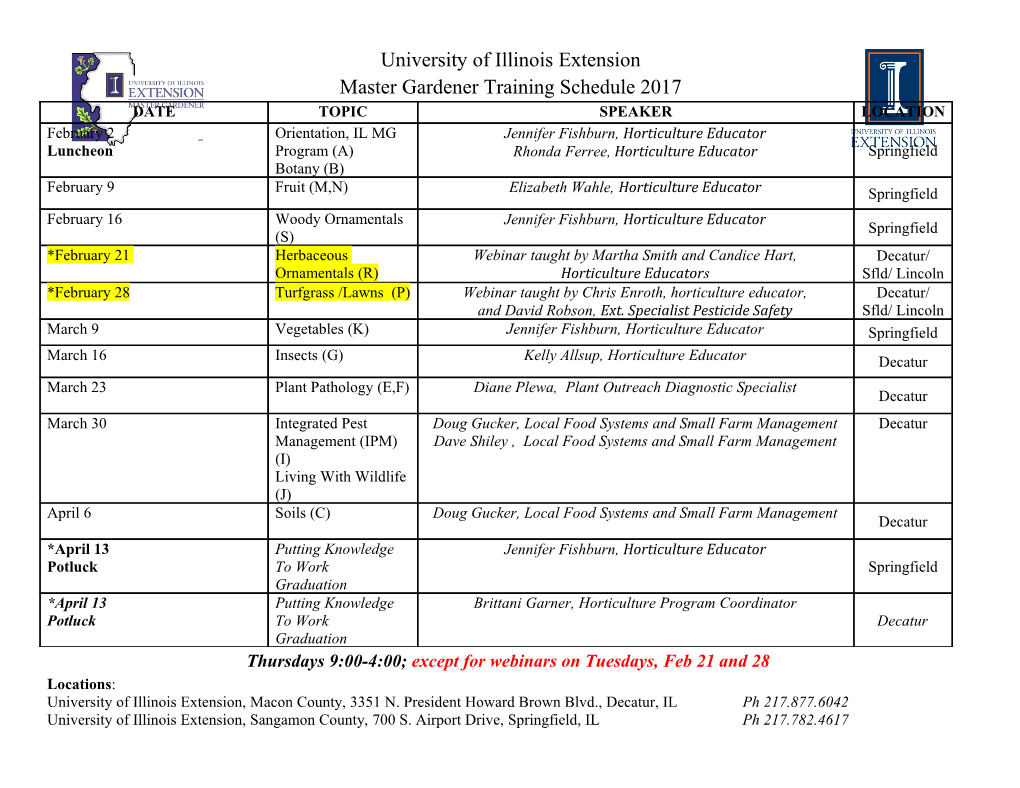
Atmospheres of hot alien Worlds Atmospheres of hot alien Worlds Proefschrift ter verkrijging van de graad van Doctor aan de Universiteit Leiden, op gezag van Rector Magnificus prof.mr. C.J.J.M. Stolker, volgens besluit van het College voor Promoties te verdedigen op donderdag 5 juni 2014 klokke 10.00 uur door Matteo Brogi geboren te Grosseto, Italië in 1983 Promotiecommissie Promotors: Prof. dr. I. A. G. Snellen Prof. dr. C. U. Keller Overige leden: Prof. dr. C. Dominik University of Amsterdam Prof. dr. K. Heng University of Bern Prof. dr. E. F. van Dishoeck Dr. M. A. Kenworthy Prof. dr. H. J. A. Röttgering Ai miei genitori, per aver creduto in me, permettendomi di realizzare un sogno Contents 1 Introduction 1 1.1 Planet properties from transits and radial velocities . 2 1.2 Atmospheric characterization of exoplanets . 4 1.2.1 Atmospheres of transiting planets . 4 1.2.2 Challenges of space- and ground-based observations . 7 1.2.3 Ground-based, high resolution spectroscopy . 8 1.2.4 A novel data analysis . 10 1.3 Close-in planets and their environment . 10 1.3.1 From atmospheric escape to catastrophic evaporation . 12 1.4 This thesis . 12 2 The orbital trail of the giant planet t Boötis b 17 2.1 The CRIRES observations . 18 2.2 Data reduction and analysis . 19 2.2.1 Initial data reduction . 19 2.2.2 Removal of telluric line contamination . 19 2.2.3 Cross correlation and signal extraction . 21 2.3 The CO detection and the planet velocity trail . 23 2.4 The planet orbit and mass . 28 2.5 The planet atmosphere . 30 2.5.1 Atmospheric models . 30 2.5.2 Comparison of model spectra to the data . 31 2.6 Discussion . 32 2.6.1 Spin-orbit alignment . 32 2.6.2 Molecular abundances and lack of an inversion layer . 32 2.7 Conclusions . 33 3 Detection of molecular absorption in the dayside of 51 Peg b? 37 3.1 Introduction . 38 3.2 Observations and Data Reduction . 40 3.2.1 Telescope and instruments . 40 3.2.2 Basic data analysis . 40 3.2.3 Stellar subtraction . 41 3.2.4 Removal of the telluric contamination . 41 3.2.5 Atmospheric models . 42 3.2.6 Cross correlation . 42 3.3 The cross-correlation signal . 45 3.4 The non-detection on the third night . 48 3.4.1 Issues with instrument, data and data analysis . 48 3.4.2 Astrophysical causes . 50 3.5 Characterization of exoplanet 51 Peg b . 51 i 3.5.1 Planet mass and system inclination . 51 3.5.2 The atmosphere of 51 Pegasi b . 51 3.6 Conclusions and future prospects . 54 4 CO and H2O in the atmosphere of the non-transiting planet HD 179949 b 59 4.1 Introduction . 60 4.2 The system HD 179949 . 61 4.3 Observations and data analysis . 62 4.3.1 Telescope, instrument and observations . 62 4.3.2 Extraction of the one-dimensional spectra . 62 4.3.3 Bad-pixel correction and wavelength calibration . 63 4.3.4 Removal of telluric lines . 64 4.4 Extraction of the planet signal . 64 4.4.1 Model spectra . 66 4.4.2 Cross correlation and signal retrieval . 67 4.4.3 Degeneracies in the atmospheric models . 68 4.5 Results . 69 4.5.1 Single-molecule detections . 69 4.5.2 Planet mass and orbital inclination . 70 4.5.3 Statistical tests on the measured signal . 72 4.5.4 Constraints on the C/O ratio . 73 4.6 Discussion and future prospects . 76 5 Evidence for the disintegration of KIC 12557548 b 83 5.1 Introduction . 84 5.2 Analysis of Kepler data . 84 5.2.1 Transit light curve . 86 5.3 The dust model . 88 5.4 Results and discussion . 90 5.4.1 Dependence on depth . 91 5.4.2 Constraints on particle size . 91 5.4.3 The size of the parent body . 92 5.5 Conclusions . 92 6 A search for rotation and atmospheric circulation in HD 189733 b 93 6.1 Introduction . 94 6.2 Observations . 96 6.3 Data analysis . 97 6.3.1 Extraction of the one-dimensional spectra . 97 6.3.2 Correction of bad-pixels and odd-even effect . 97 6.3.3 Alignment of spectra and wavelength calibration . 98 6.3.4 Removal of the stellar spectrum . 99 6.3.5 Removal of telluric lines . 100 6.4 Modeling and extracting the planet signal . 101 6.4.1 Modeling the planet spectra . 101 6.4.2 Modeling the planet broadening profile . 102 ii 6.4.3 Cross correlation and signal retrieval . 104 6.5 Results . 106 6.5.1 Planet signal from models with single trace gases . 106 6.5.2 Planet signal from models with all molecular species . 107 6.5.3 Impact of Sysrem algorithm on the measured signal . 109 6.5.4 Absence of signal in the partially-observed transit . 111 6.6 Discussion . 111 6.A Modeling and removal of the stellar spectrum . 115 6.A.1 Creating the telluric spectrum . 115 6.A.2 Creating the stellar spectrum . 115 6.A.3 Fitting the CO lines . 116 6.A.4 Subtracting the CO lines . 116 7 Future exoplanet characterization at high spectral resolution 117 7.1 Advantages of high-resolution spectroscopy . 117 7.2 Current limits of high-resolution spectroscopy . 118 7.3 The coming years . 119 7.4 The next decade: Extremely Large Telescopes . 121 7.5 Final remarks . 123 Samenvatting 125 Riepilogo 129 List of publications 133 Curriculum Vitae 135 Acknowledgments 137 iii 1 Introduction The origin of planetary systems and the existence of life elsewhere in the Universe are arguably among the most fascinating subjects of modern astrophysics. For cen- turies mankind could only speculate about the existence of planets orbiting stars other than the Sun (exoplanets), and even in relatively modern times the technolog- ical challenges for discovering such bodies were too overwhelming. The last two decades revolutionized the field of exoplanets, so that we are currently living the golden era of their discoveries. Thanks to dedicated surveys and telescopes, oper- ating both in space and from the ground, planet hunters have found more than a thousand objects1, and a sample three times bigger awaits confirmation. Perhaps the most surprising characteristic of the known exoplanets is their diver- sity, which has no analogs in the Solar System. Figure 1.1 shows the known planets to date in a semi-major axis, planet mass diagram (a, MP). The top-left corner of the diagram pictures a population of giant planets in very close orbits, much closer to their parent star than Mercury is to the Sun, which are called hot Jupiters. The existence of this population challenged the main-stream theories of planet forma- tion and evolution at the time of the first discoveries (Mayor & Queloz 1995; Marcy & Butler 1996; Butler et al. 1997), and led to the introduction of planet migration during the early stages of planet formation (Lin et al. 1996; Rasio et al. 1996). A more recent surprise was the discovery of bodies intermediate between the size of the Earth and that of Uranus. Despite no such object is observed around the Sun, they seem to be the most common planets in our Galaxy (Fressin et al. 2013; Petigura et al. 2013). The two examples above suggest that, in the general picture of planet formation and evolution, our Solar System is uncommon, and must be placed in context by deriving the properties of exoplanets as accurately as possible. These include their masses and radii, the orbital parameters, and the atmospheric structure and com- position. Studying exoplanet atmospheres is a crucial task for identifying habitable planets and detecting possible signatures of life, which is one of the ultimate goals of exoplanet sciences. Up to now, the majority of the exoplanet detections have been achieved by using two indirect methods, namely the radial velocity and transit techniques. The direct detection of a planet, which implies imaging the light of the planet separately from that of the host star, is instead a challenging task, which has only recently been demonstrated to be successful (Chauvin et al. 2005; Kalas et al. 2008; Marois et al. 2008). It is so far limited to young, self-luminous planets in large orbits (tens to hun- dreds of astronomical units), although state-of-the-art instruments such as SPHERE at the ESO Very Large Telescope (Beuzit et al. 2006) or the Gemini Planet Imager 1Source: exoplanet.eu 1 2 1. Introduction 10 ) Jup 1 10-1 Radial velocities Planet mass (M 10-2 Transits Direct Imaging Other 10-3 10-3 10-2 10-1 1 10 102 103 Semi-major axis (AU) Figure 1.1: The known exoplanets to date in a semimajor axis-mass (a, MP) diagram. The main discovery techniques are labeled with different colors. We note how tran- sits and radial velocities are complementary in the mass range of giant planets, and how direct imaging is currently limited to massive planets on large orbits. (Macintosh et al. 2006) will push the detection threshold towards more evolved sys- tems and/or smaller orbits. For the remainder of this introductory Chapter, we will focus on the indirect methods for discovering and characterizing exoplanets. 1.1 Planet properties from transits and radial velocities Due to the fact that a star and planet both orbit their common center of mass, the presence of the planet can be inferred from the periodic Doppler shift of the stellar spectral lines.
Details
-
File Typepdf
-
Upload Time-
-
Content LanguagesEnglish
-
Upload UserAnonymous/Not logged-in
-
File Pages148 Page
-
File Size-