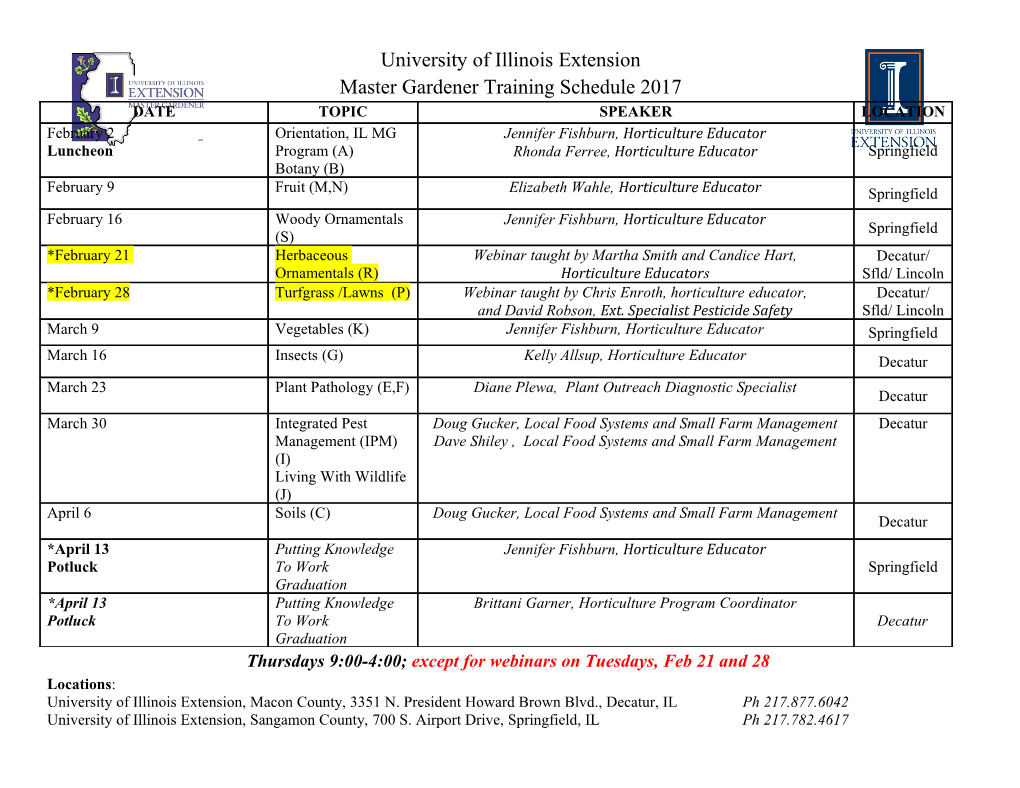
THE LEACHING AND PASSIVATION OF CHALCOPYRITE IN ACID SULFATE MEDIA by RALPH PETER HACKL B.Sc.(Hons.), The University of British Columbia, 1977 M.Sc, The University of British Columbia, 1983 A THESIS SUBMITTED IN PARTIAL FULFILLMENT OF THE REQUIREMENTS FOR THE DEGREE OF DOCTOR OF PHILOSOPHY in THE FACULTY OF GRADUATE STUDIES Department of Metals and Materials Engineering We accept this thesis as conforming to the required standard THE UNIVERSITY OF BRITISH COLUMBIA November 1995 © Ralph Peter Hackl, 1995 In presenting this thesis in partial fulfillment of the requirements for an advanced degree at the University of British Columbia, I agree that the library shall make it freely available for reference and study. I further agree that permission for extensive copying of this thesis for scholarly purposes may be granted by the head of my department or by his or her representatives. It is understood that copying or publication of this thesis for financial gain shall not be allowed without my written permission. Department of Metals and Materials Engineering The University of British Columbia Vancouver, B.C., Canada Date: HoVe^bvr 20, 199$ ABSTRACT Sulfate-based leaching processes for chalcopyrite (CuFeSa) are attractive because of their inherent simplicity. Unfortunately, high copper extractions are not attainable in a reasonable residence time unless the leaching temperature exceeds 200°C (oxygen pressure leaching) or chalcopyrite is "activated" by a pretreatment method prior to leaching. In the present work, the oxygen pressure leaching behaviour of chalcopyrite in the temperature range 110-220°C was studied to shed new light on the reasons for the slow leaching. At temperatures where molten elemental sulfur is stable (120-180°C), the reaction was found to be prematurely stifled by liquid sulfur formed during leaching. The liquid sulfur wetted and agglomerated the unreacted mineral particles, effectively stopping the leach at 40-70% copper extraction. The feasibility of using surfactants to disperse the sulfur and enhance the copper extraction in the temperature range 125-155°C was investigated. Most of the surfactants tested decomposed too rapidly to be of benefit. The best results were obtained with orthophenylenediamine (OPD) when it was added continuously at a low temperature (125°C) and a high dosage (50 kg/t). Under these conditions the surfactant was successful in dispersing liquid sulfur and it effected a modest increase in copper extraction over that obtained at 110°C, but only after prolonged retention times (6 hours). It was found that chalcopyrite leached slowly even if molten sulfur was prevented from wetting the mineral surfaces. This led to the conclusion that the reaction rate is ultimately controlled by a passivating mechanism unrelated to elemental sulfur formation. Oxidized chalcopyrite mineral surfaces were examined by Auger electron spectroscopy and X-ray photoelectron spectroscopy to identify the presence of any passivating layers that may form during leaching. The results indicated that an iron-deficient, copper-rich sulfide layer forms on the surface of chalcopyrite as a result of solid state changes that occur in the mineral during leaching. This layer is thought to be a copper polysulfide, CuSn, where n > 2. The layer is quite thin (-10-100 nm) and hence is not detectable by conventional electron microscopy. The copper polysulfide is believed to passivate the chalcopyrite. The addition of silver ions at a temperature below the sulfur melting point (110°C) was found to catalyze the leach, resulting in the copper extraction increasing from 53% to 96% after -111- 3 hours leaching. Silver alone was ineffective at 125°C and 155°C because the leach was stifled by liquid sulfur. However, when silver was used in conjunction with OPD at 125°C the copper extraction was again enhanced significantly, to 91%. The OPD prevented the sulfur from wetting the mineral surfaces, allowing the catalytic effect of the silver ions to be maintained. A simple mathematical model was formulated to explain the leaching and passivation of chalcopyrite at low temperature (110°C). The reaction kinetics can be explained in terms of a mixed diffusion/chemical reaction model where the reaction rate is initially dependent on the rate at which copper and iron diffuse through the thickening passive layer. The passive layer also leaches, but at a slower rate than the chalcopyrite. Eventually, the passive layer reaches a steady state thickness and the kinetics are then controlled by the rate at which the passivating layer decomposes. The linear rate constant k\ for the decomposition of the passive layer was calculated to be 5.0x10"3 um/min. The low value of k\ explains why inordinately long residence times are required to obtain a high copper extraction from chalcopyrite at 110°C. -iv- TABLE OF CONTENTS ABSTRACT ii TABLE OF CONTENTS iv List of Tables viii List of Figures x List of Symbols xiv ACKNOWLEDGEMENTS xvii CHAPTER 1 - INTRODUCTION 1 CHAPTER 2 - LITERATURE REVIEW 4 2.1 Elemental Sulfur 4 2.1.1 Properties of Elemental Sulfur Allotropes 4 2.1.2 Aqueous Oxidation of Elemental Sulfur in Acid Sulfate Media 6 2.2 Properties of Chalcopyrite 10 2.2.1 Crystal Structure 10 2.2.2 Thermodynamic Considerations 11 2.3 Leaching of Chalcopyrite in Sulfate Media 14 2.3.1 Ferric Sulfate Leaching 14 2.3.2 Oxygen Pressure Leaching 16 2.3.3 Bioleaching 19 2.4 Passivation of Chalcopyrite 22 2.4.1 Electrochemical Studies 22 2.4.2 Surface Studies 26 2.5 Activation of Chalcopyrite 29 2.5.1 Thermal Activation 29 2.5.2 Mechanical Activation 31 2.5.3 Chemical Activation with Silver Ions 32 2.6 Use of Sulfur Dispersing Surfactants in Leaching 34 2.6.1 Overview of Surfactant Properties in Mineral-Aqueous Media 34 2.6.2 Surfactants in Oxygen Pressure Leaching of Zinc Concentrates 38 2.6.3 Surfactants in Oxygen Pressure Leaching of Nickeliferous Pyrrhotite Concentrates 40 2.6.4 Surfactants in Pressure Oxidation of Refractory Gold Ores and Concentrates 42 2.7 Conclusions and Focus of the Present Study 44 CHAPTER 3 - BASIC PRINCIPLES OF AUGER ELECTRON SPECTROSCOPY AND X-RAY PHOTOELECTRON SPECTROSCOPY 46 3.1 Introduction 46 3.2 Notation used in AES and XPS 47 3.3 Auger Electron Spectroscopy 47 3.3.1 The Auger Process 47 3.3.2 Properties of Auger Electrons 49 -V- 3.3.3 Generation and Interpretation of Auger Spectra 50 3.3.4 Strengths and Limitations of AES 51 3.4 X-ray Photoelectron Spectroscopy 52 3.4.1 The XPS Process 52 3.4.2 Properties of Photoelectrons 53 3.4.3 Generation and Interpretation of XPS Spectra 53 3.4.3.1 Core Level Emission Peaks 54 3.4.3.2 X-ray-Excited Auger Emission Peaks 55 3.4.3.3 Secondary Peaks 56 3.4.3.4 Quantification 57 3.4.4 Strengths and Limitations of XPS 57 3.5 Sputter Depth Profiling 57 CHAPTER 4 - EXPERIMENTAL PROCEDURES 59 4.1 Interfacial Experiments 59 4.1.1 Reagents 59 4.1.2 Surfactants 59 4.1.3 Chalcopyrite Mineral 61 4.1.4 Apparatus 61 4.1.5 Formation of Pendant Drops 63 4.1.6 Formation of Sessile Drops 64 4.1.7 Determination of the Aqueous Solution Density at Elevated Temperature 65 4.1.8 Determination of Interfacial Tensions and Contact Angles 66 4.1.8.1 Interfacial Tensions by the Method of the Selected Plane 66 4.1.8.2 Manual Measurement of Contact Angles 67 4.1.8.3 Axisymmetric Drop Shape Analysis 68 4.1.9 Determination of Work of Adhesion Values 69 4.2 Oxygen Pressure Leaching Experiments 69 4.2.1 Reagents 69 4.2.2 Mineral Samples 70 4.2.2.1 Gibraltar Copper Concentrate 70 4.2.2.2 Messina Chalcopyrite 70 4.2.2.3 Covellite 71 4.2.3 Particle Size Analyses of the Mineral Samples 72 4.2.3.1 Sieve Analyses 72 4.2.3.2 Particle Size Analysis with the Elzone Particle Size Counter 73 4.2.3.3 Particle Size Analysis with the Horiba Photosedimentation Instrument.. 73 4.2.4 Surfactants , 74 4.2.5 Pressure Leaching Apparatus and Procedures 74 4.2.6 Analytical Methods 77 4.2.7 Calculation of Metal Extractions and Sulfide Oxidation Extents 78 4.3 Analysis of Chalcopyrite Surfaces 79 4.3.1 Sample Preparation 79 4.3.2 Auger Electron Spectroscopy Procedures 80 4.3.3 X-ray Photoelectron Spectroscopy Procedures 80 CHAPTER 5 - RESULTS AND DISCUSSION 82 5.1 Interfacial Experiments 82 -vi- 5.1.1 Effect of Surfactants on the Sulfur-Solution Interfacial Tension 82 5.1.2 Effect of Surfactants on the Sulfur-Chalcopyrite Contact Angle....'. 83 5.1.3 Effect of Surfactants on the Work of Adhesion 86 5.1.4 Discussion 87 5.1.4.1 Surfactants and Sulfur-Solution Interfacial Tensions 87 5.1.4.2 Surfactants and Sulfur-Chalcopyrite Contact Angles 88 5.1.4.3 Possible Surfactant-Chalcopyrite and Surfactant-Sulfur Bonding Interactions 90 5.2 Pressure Leach Experiments 91 5.2.1 Effect of Temperature on Chalcopyrite Reaction Kinetics and Sulfur Yield .... 91 5.2.2 Effect of Liquid Sulfur on Chalcopyrite Oxidation 96 5.2.3 Preliminary Evaluation of Surfactants in Pressure Leaching Tests 103 5.2.4 Effect of Continuous Surfactant Addition at High Pulp Density 105 5.2.5 Effect of Batch and Continuous Surfactant Additions at Low Pulp Density... 106 5.2.6 Effect of Surfactants In Silver Catalyzed Oxygen Pressure Leaching 108 5.2.6.1 Effect of Silver in the Absence of Surfactants 110 5.2.6.2 Effect of Silver in the Presence of Surfactants 113 5.2.7 Discussion 115 5.3 Surface Characterization Experiments 117 5.3.1 Auger Electron Spectroscopy Results 117 5.3.1.1 Spectral Features 117 5.3.1.2 Surface Composition Results 119 5.3.1.3 Sputter Depth Profiling Results 120 5.3.2 X-ray Photoelectron Spectroscopy Results 123 5.3.2.1 GeneralFeatures of Broad-Scan Spectra 123 5.3.2.2
Details
-
File Typepdf
-
Upload Time-
-
Content LanguagesEnglish
-
Upload UserAnonymous/Not logged-in
-
File Pages211 Page
-
File Size-