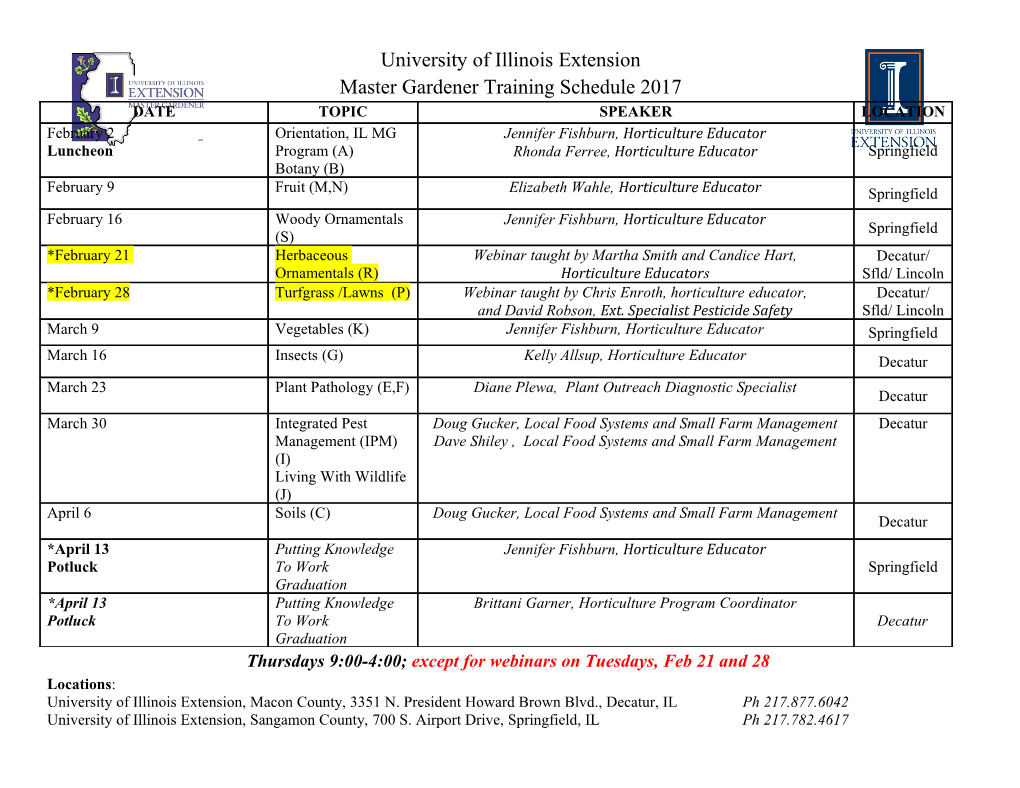
CHAPTER 7 GLACIERS 1. INTRODUCTION 1.1 Before the era of universal air travel, which commenced less than half a century ago, few of the world’s population had seen a glacier. I suspect that majority of class members in this course have seen a glacier—if not close up, then out of a jetliner window. In the Canadian Rockies, you can drive to within almost a stone’s throw of the terminus of the Athabasca Glacier, a classic active valley glacier. In many other parts of the world, valley glaciers are accessible to even casual day hikers. The great ice sheets of the world, in Antarctica and Greenland, remain much less accessible. 1.2 In the broad context of geologic history, the Earth is in an “icehouse” time, with recurrent major ice-sheet advances across the Northern Hemisphere continents. (There have been several other such icehouse periods in Earth history, separated by long intervals of ice-free times, called “greenhouse” periods, with no evidence of glaciation.) The Earth has only recently emerged from the latest episode of continental glaciation. Does it surprise you to learn that a mere twenty thousand years ago the Boston area was beneath a mile of glacier ice moving slowly southward toward its terminus south of what is now the south coast of New England? 1.3 The Earth is in many senses a glacial planet: • 10% of the Earth is covered with glacier ice (about 15 million square kilometers). • About 40% of the Northern Hemisphere in winter is covered with solid water at any given time (land and sea). • 75% of the Earth’s fresh water is in glaciers. • Surficial deposits by glaciers cover a large percentage of the Earth’s land surface. • Glaciers have a profound effect on the Earth’s climate (as well as being in turn controlled by climate). 1.4 And glaciers are not without practical importance: • Role of ice sheets on climate • Control on sea level 284 • Potential source of fresh water 1.5 Disciplines: in the US, people who deal with glaciers or their products are usually allied to geology; in other English-speaking countries, usually to geography. Disciplines relevant to this chapter: glaciology glacial hydrology glacial geology geomorphology sedimentology stratigraphy 1.6 What is a glacier?: a body of ice and recrystallized snow (plus refrozen meltwater), on land (or, if floating, then connected to land) and moving by deformation under its own weight. 1.7 Why are there glaciers? A necessary and sufficient condition is an excess of snowfall over snowmelt at some locality for a long enough time to build up ice thick enough for it to flow under its own weight. 2. CLASSIFICATION OF GLACIERS 2.1 One good way of classifying glaciers is by the extent to which their shape and movement are affected by the underlying bedrock topography. Glaciers not strongly constrained by underlying topography: Ice sheet: Superimposed on underlying topography; largely or entirely submerges that topography; ice flow reflects largely the size and shape of the glacier, less the shape of the ground. Ice sheets smaller than about 50,000 km2 in area are called ice caps. Ice dome: About symmetrically over land area involved; the top of the dome may be over bedrock highs or bedrock lows. Highest: 4300 m, Antarctica. The convex profile is a reflection mainly of flow mechanics, including the nature of the bottom roughness. 285 Outlet glacier: A stream of ice that extends beyond an ice dome and drains it. Outlet glaciers are often very large: several hundred kilometers long and tens of kilometers wide. Commonly they are commonly associated both with large ice sheets and with smaller ice caps. Most of the Greenland ice sheet and about three-quarters of the Antarctic ice sheet is drained in this way. Ice shelf: A floating ice sheet that deforms under its own weight. The slab of ice is squeezed between the atmosphere and the ocean. The ice shelf has to be anchored at several points. In Antarctica, ice shelves constitute about 7% of ice area. The ice shelf may be fed partly or not at all by land glaciers. Accumulation: snow on the surface; land glaciers; bottom freezing. Ablation: melting (at the top or the bottom); calving. Glaciers strongly constrained by topography: Ice field: An approximately level area of ice, not an ice cap because not domelike; the flow reflects the underlying bedrock topography (ice fields are usually nestled in among mountains). Size: a few square kilometers to very large. Ice fields grade over into small ice caps. Requirement for existence of an ice field: high and overall gentle topography. Valley glacier: A glacier flowing in a rock valley and surrounded by rock walls (therefore long and linear). Fed from an ice field or a cirque (see below). Usually 10–30 km long, but up to 100 km. The terminus can be on land or in the sea (by calving). A valley glacier can also disgorge from mountains and spread out as a flat mass in piedmont areas to form a what is called a piedmont glacier. A good example of a piedmont glacier: the Malaspina Glacier, in Alaska, 40 km across. Valley glaciers are usually vigorous glaciers. And because they are typically present at low as well as high latitudes, they are among the most accessible of glaciers. Cirque glacier: A small ice mass, fairly wide relative to its length, occupying a bedrock hollow or basin, usually on a mountain slope. 286 3. DISTRIBUTION OF GLACIERS 3.1 The total area of the Earth’s surface covered by glaciers is 14.9 million km2 • Antarctic ice sheet: 12.5 million km2 • Greenland ice sheet: 1.7 million km2 • All the rest: 700,000 km2 (many ice caps, mostly less than about 10,000 km2; many thousands of small glaciers, mostly valley glaciers) 3.1 Aside from the Greenland ice sheet, most of the larger glaciers in the Northern Hemisphere are mostly on Iceland and the Arctic Islands of Canada, because of the distribution of land and sea. 3.2 It’s a lot more difficult to get the volumes of ice in glaciers than to get the areas covered by glaciers. Things have gotten better in this respect, though, because of use of echo sounding, similar to oceanographic depth sounding. But one still has to go out there to the glacier to do it. Antarctica: 21.5 million km3 (sea-level equivalent about 60 m) Greenland: 2.4 million km3 (sea-level equivalent about 5 m) All other: 180,000 km3 (largest ice cap: Vatnajökull, Iceland, 3100 km3, and fairly accessible). 4. GLACIER ICE 4.1 The transition of new snow to glacier ice is similar to the deposition, diagenesis, and metamorphism of a sediment to form a metamorphic rock. (And in a real sense, glacier ice is a metamorphic rock.) 4.2 New dry snow has low bulk density and high porosity, and enormous internal surface area. Crushing, compaction, and exchange of water between flakes and air at low temperatures by sublimation and deposition, and at temperatures near melting by melting, refreezing, evaporation, and condensation, tend to round grains, producing the nearly spherical grains of granular snow. Except in the coldest environments, this takes a few days to a few weeks. In the process, the snow settles and gets more compact, and small grains disappear at the expense of larger ones. The recrystallized granular snow, still friable and porous, is called firn when it is more than a year old. The firn is then converted to glacier ice as compaction due to increasing weight of overlying younger snow continues. Air is forced out, decreasing the intergranular space. Recrystallization proceeds by solution at points of contact and deposition in the interspaces. 287 4.3 When the permeability to air has reached almost zero, the firn has become glacier ice. This change occurs in a relatively narrow density range around 0.82–0.84 g/cm3, at depths of greater than 30 m. Crystal size has increased to about 1 cm. Firn is transformed into glacier ice in one year to a few hundred years, depending on temperature and rate of accumulation. Then, depending on both temperature and bottom slope, something like 50 m of ice is needed for the ice to flow plastically under its own weight (there’s more detail on flow of glacier ice in a later section). The ultimate density of glacier ice approaches 0.90 g/cm3, a bit short of the density of 0.917 g/cm3 of pure ice because of the inevitable residual presence of little air bubbles. 5. THE BUDGET OF GLACIERS 5.1 Economy vs. Regimen 5.1.1 First off, the term accumulation applies to all of the ways glacier ice mass is added to a glacier, and the term ablation applies to all of the ways glacier ice is removed from a glacier. 5.1.2 The term economy refers to the relative magnitude of accumulation and ablation. A glacier with accumulation greater than ablation over some period of time (much longer than just a single year) is said to have a positive economy, and gains ice volume with time. A glacier with accumulation less than ablation is said to have a negative economy, and loses ice volume with time. A glacier with a positive economy not only thickens but also extends its terminus in the downglacier direction. A glacier with a negative economy becomes thinner, and the terminus retreats upglacier. 5.1.3 The term regimen refers to the absolute values or magnitudes of accumulation and ablation, irrespective of their balance.
Details
-
File Typepdf
-
Upload Time-
-
Content LanguagesEnglish
-
Upload UserAnonymous/Not logged-in
-
File Pages81 Page
-
File Size-