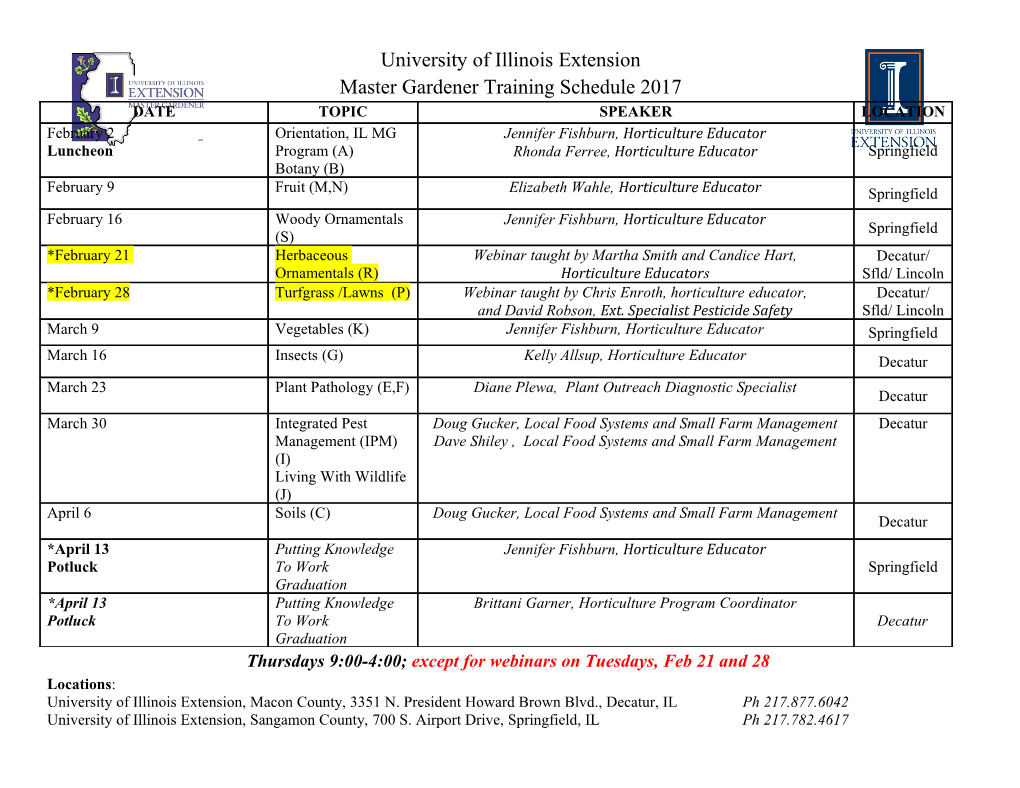
LETTER doi:10.1038/nature11692 An alternative route to cyclic terpenes by reductive cyclization in iridoid biosynthesis Fernando Geu-Flores1, Nathaniel H. Sherden1, Vincent Courdavault2, Vincent Burlat3,4, Weslee S. Glenn1,5, Cen Wu6, Ezekiel Nims5{, Yuehua Cui6 & Sarah E. O’Connor1,7 The iridoids comprise a large family of distinctive bicyclic mono- step via a Diels–Alder cycloaddition or a Michael addition. Our terpenes that possess a wide range of pharmacological activities, results illustrate how a short-chain reductase was recruited as including anticancer, anti-inflammatory, antifungal and anti- cyclase for the production of iridoids in medicinal plants. Further- bacterial activities1–4. Additionally, certain iridoids are used as sex more, we highlight the prospects of using unrelated reductases to pheromones in agriculturally important species of aphids, a fact generate artificial cyclic scaffolds. Beyond the recognition of an that has underpinned innovative and integrated pest management alternative biochemical mechanism for the biosynthesis of cyclic strategies5. To harness the biotechnological potential of this natural terpenes, we anticipate that our work will enable the large-scale product class, the enzymes involved in the biosynthetic pathway heterologous production of iridoids in plants and microorganisms must be elucidated. Here we report the discovery of iridoid synthase, for agricultural5–8 and pharmaceutical1–4,9 applications. a plant-derived enzyme that generates the iridoid ring scaffold, as The crucial step in the biosynthesis of all cyclic terpenes is the evidenced by biochemical assays, gene silencing, co-expression ana- cyclization step that gives rise to the individual ring systems. In all lysis and localization studies. In contrast to all known monoterpene kingdoms of life, this step has been shown to be catalysed by terpene cyclases, which use geranyl diphosphate as substrate and invoke a cyclases, which are terpene synthases that use polyprenyl diphosphates cationic intermediate, iridoid synthase uses the linear monoter- such as geranyl diphosphate to produce a cationic species that is sub- pene 10-oxogeranial as substrate and probably couples an initial sequently cyclized and rearranged to form one of hundreds of possible NAD(P)H-dependent reduction step with a subsequent cyclization ring structures10,11. However, the cyclization step that leads to the Glc a HO O b Glc H O 9 O OH O O O O H Iridoid HO O 2 H H H H 3 1 synthase O O O O O O 4 7 NAD(P)H + HO H 10 H 5 6 H Catalpol H H HO H 8 Aucubin Cis-trans- 10-oxogeranial Cis-trans- Cis-trans- nepetalactone nepetalactol iridodials c d e 13 TLC, anisaldehyde stain GC–MS, TIC C-NMR Minus NADPH Isolated product 10-oxogeranial 3 Plus NADPH CDCl Cis-trans Cis-trans-nepetalactol -nepetalactol Intensity Enzyme +–+–+ Intensity 3 NADPH –++–– Cis-trans-nepetalactol, CDCl NADH –––++ Cis-trans- extracted from water nepetalactol 10-oxogeranial 150 125 100 75 50 25 0 8.00 8.50 9.00 9.50 10.00 10.50 11.00 11.50 Chemical shift (p.p.m.) Time (min) Figure 1 | Identification of the iridoid synthase from C. roseus.a, Chemical chromatograms (TICs) of CH2Cl2 extracts of the iridoid synthase reaction (‘plus structure of three plant iridoids. Glc, b-glucopyranosyl. b, The iridoid synthase NADPH’) and of a negative control (‘minus NADPH’) are compared to the TICs reaction in C. roseus (products may possessalternative stereochemistries in other of freshly synthesized cis-trans-nepetalactol or cis-trans-nepetalactol that had organisms). c, Analysis of the reaction by TLC. CH2Cl2 extracts of different been dissolved in water and subsequently extracted with CH2Cl2. e,Comparison reaction mixtures were run in parallel with authentic samples of the substrate of the 13C-NMR spectra of the purified major product from a milligram-scale and expected product. d, Analysis of the reaction product by GC–MS. Total ion enzymatic reaction and the synthetic cis-trans-nepetalactol. 1Department of Biological Chemistry, John Innes Centre, Norwich NR4 7UH, UK. 2Universite´ François-Rabelais de Tours, EA2106, Biomole´cules et Biotechnologies Ve´ge´tales, 37200 Tours, France. 3Universite´ de Toulouse, UPS, UMR 5546, Laboratoire de Recherche en Sciences Ve´ge´tales, BP 42617 Auzeville, F-31326 Castanet-Tolosan, France. 4CNRS, UMR 5546, BP 42617, F-31326 Castanet- Tolosan, France. 5Department of Chemistry, Massachusetts Institute of Technology, Cambridge, Massachusetts 02139, USA. 6Department of Statistics and Probability, Michigan State University, East Lansing, Michigan 48824, USA. 7School of Chemistry, University of East Anglia, Norwich NR4 7TJ, UK. {Present address: Ra Pharmaceuticals, Inc., Cambridge, Massachusetts 02139, USA. 138 | NATURE | VOL 492 | 6 DECEMBER 2012 ©2012 Macmillan Publishers Limited. All rights reserved LETTER RESEARCH characteristic 5–6 bicyclic scaffold of iridoids (Fig. 1a) is markedly NADPH-using enzymes showed high similarity to progesterone-5b- different. Feeding studies have suggested that the direct precursor of reductase (P5bR) (67% amino acid identity compared to Digitalis all iridoids in plants is not geranyl diphosphate, but the linear mono- purpurea P5bR2) (Supplementary Fig. 1a–c), an enzyme that reduces terpene 10-oxogeranial12–15. These studies also suggest that the bicyclic the C–C double bond of the a,b-unsaturated carbonyl of progesterone compound nepetalactol is the general precursor of the more deri- in cardenolide biosynthesis18. Interestingly, many plant species, including vatized iridoids. Assays using crude plant extracts have indicated that C. roseus, possess P5bR homologues but do not produce cardenolides, 10-oxogeranial can be converted to nepetalactol in a NADH/NADPH- indicating that numerous genes annotated as P5bR have some other dependent manner (Fig. 1b)16. Nevertheless, the molecular identity physiological function19. of the enzyme catalysing this reaction, which we here call iridoid We expressed the iridoid synthase candidate in Escherichia coli, and synthase, has hitherto remained unknown. although the heterologous enzyme did not metabolize progesterone, To identify iridoid synthase, we used recently available transcrip- analysis by thin-layer chromatography (TLC) showed that it metabo- tomic data from Catharanthus roseus (Apocynaceae)17, a medicinal lized 10-oxogeranial in the presence of either NADH or NADPH plant that produces a variety of iridoid-derived monoterpene indole (Fig. 1c). The identity of the product was confirmed by gas chromato- alkaloids (MIAs), including the anticancer alkaloid vinblastine. graphy–mass spectrometry (GC–MS) analysis, which also evidenced Because hundreds of NAD(P)H-dependent enzymes are encoded in the expected equilibrium between nepetalactol and its open dialdehyde the C. roseus transcriptome, we first applied a co-regulation criterion forms (iridodials) in water (Fig. 1d). A milligram-scale enzyme reaction to reduce the number of candidates, reasoning that the iridoid synthase allowed the NMR-based assignment of the purified product as cis- would probably have an expression profile similar to geraniol 10- trans-nepetalactol (Fig. 1e, see nomenclature in ref. 20), which is the hydroxylase (G10H), the closest characterized enzyme upstream of expected isomer. The steady-state kinetic constants of the reaction the cyclization step. In addition to a number of transcripts likely to be involved in the biosynthesis of MIAs, we observed two transcripts O O a O + coding for NADPH-using enzymes among the 20 best co-regulated H– H H H O O O transcripts (Supplementary Table 1). The higher-ranking of these two or H H N H b O a EV control (n = 6) O VIGS iridoid OAc 1: 10-oxogeranial 1.6 synthase (n = 7) MeO O 2: citral (geranial + neral) ) N CO2Me 1.4 10 2.5 MeHOH 3: 6,7-dihydro-10-oxogeranial R 10 4: ( )-10-oxocitronellal 1.2 × Vindoline 100 2.0 5: 10-oxogeraniol 1.0 6: (S)-10-oxocitronellal 1.5 EV control (n = 6) S 0.8 7: ( )-citronellal VIGS iridoid 80 8: (R)-citronellal 0.6 1.0 synthase (n = 7) * Normalized 0.4 relative expression 0.5 0.2 Normalized intensity * 60 0.0 (peak area/leaf mass 0.0 O G10H LAMT Iridoid OH O O synthase O S Vindoline 40 ( ) Strictosidine O Catharanthine (R) O O b c O G10H AS 20 (S) IPAP O (R) Relative NADPH consumption rate (%) LC–MS, EIC (nine selected masses) VIGS (EV control vs iridoid synthase) 0 2 9 12345678 12+20 7 3+17 Iridoid synthase AS IPAP Compound number 5 13 c OH Intensity In vivo feeding (mock vs 10-oxogeranial) Diels–Alder 9 (1) O 7 + 3+17 H 12+20 2 OH 5 13 Iridoid synthase S O H– Cis-trans- O O nepetalactol/iridodial 1 2 3 4 5 6 7 8 in equilibrium Time (min) OH H+ 100 μm (2) O Michael addition Figure 2 | In vivo studies of iridoid synthase. a, Transcript downregulation by VIGS. Left, relative transcript quantification by real-time PCR, including data for two known iridoid-related enzymes, G10H and loganic acid Figure 3 | Substrate specificity and mechanistic considerations. methyltransferase (LAMT). Right, quantification of the three major a, Hypothetical fully concerted mechanism, which yields products with different monoterpene indole alkaloids (MIAs) by LC–MS. EV, empty vector control. stereochemistry than that of the cis-trans product observed experimentally Asterisks denote statistical significance (P , 0.05 on unpaired Student’s t-tests). (compare to Fig. 1a). Only one set of possible enantiomers is depicted. Error bars represent standard errors. b, LC–MS analysis of compounds b, Substrate specificity. Relative activities for the different test substrates are accumulating in both VIGS experiments (upper traces) and 10-oxogeranial expressed as percentages of the NADPH consumption rate for 10-oxogeranial. feeding experiments (lower traces), including their respective controls (black Product elucidation by GC–MS is presented in Supplementary Fig. 6 for traces). Traces are extracted ion chromatograms (EICs). Peak numbers refer to substrates 2, 3, 4 and 6. Error bars represent standard errors. c, Two proposed Supplementary Table 2.
Details
-
File Typepdf
-
Upload Time-
-
Content LanguagesEnglish
-
Upload UserAnonymous/Not logged-in
-
File Pages7 Page
-
File Size-