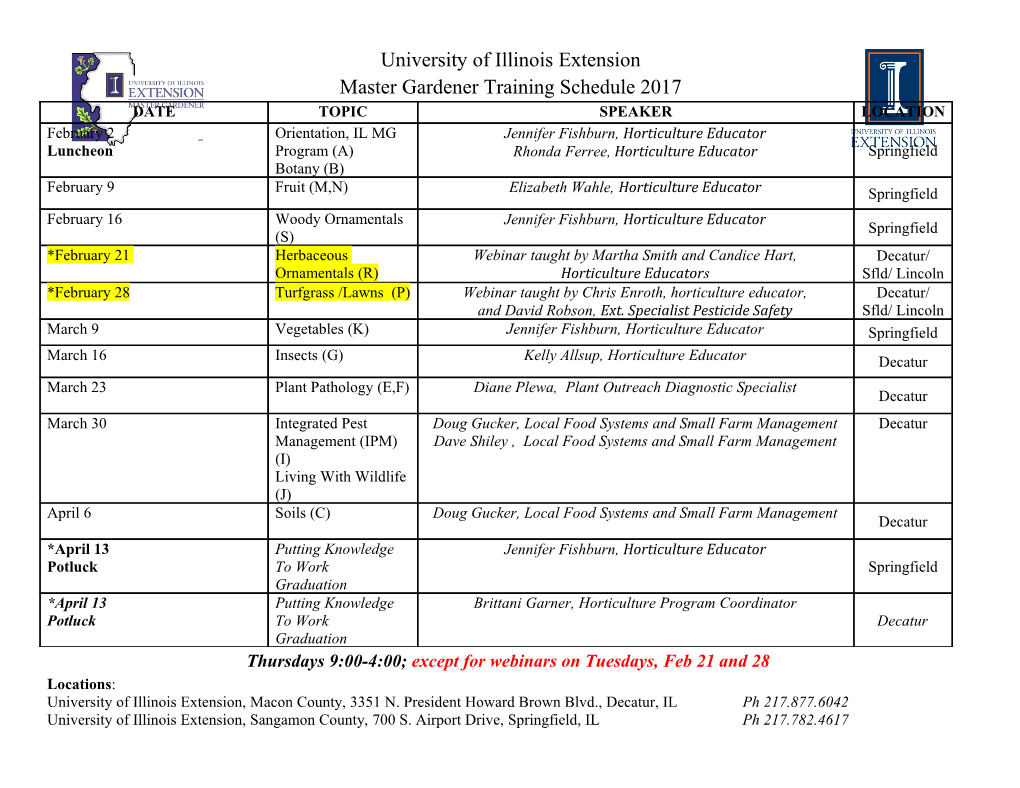
Analysis and design of Piezoelectric sonar transducers. Rodrigo, Gerard Christopher The copyright of this thesis rests with the author and no quotation from it or information derived from it may be published without the prior written consent of the author For additional information about this publication click this link. http://qmro.qmul.ac.uk/jspui/handle/123456789/1712 Information about this research object was correct at the time of download; we occasionally make corrections to records, please therefore check the published record when citing. For more information contact [email protected] -1- ANALYSIS AND DESIGN OF PIEZOELECTRIC SONAR TRANSDUCERS Gerard Christopher Rodrigo Department of Electrical and Electronic Engineering, Queen Mary College, London E.l. Thesis presented for the Degree of Doctor of Philosophy of the University of London August 1970 -2- ABSTRACT In this study techniques are developed for the analysis and design of piezoelectric sonar transducers based on equivalent circuit representations. For the purposes of analysis, equivalent circuits capable of accurately representing every element of a transducer in the full operating frequency range, are developed. The most convenient fashion in which these equivalents could be derived is also discussed. For the purposes of design the accurate equivalents are approximated by L-C-R circuits. The limits of both representations are discussed in detail. The technique of analysis developed is capable of determining the frequency characteristics as well as the transient response to any electrical or acoustic input which can be specified analytically or numerically in the time domain. The design technique is based on the formulation of a ladder-type generalized circuit incorporating the essential components of any transducer. The generalised circuit is then used to extract particular bandpass filter designs which possess wide pasabands and which are wechanically realizable. By this procedure it is found possible to design transducers exhibiting bandwidths of around 100%. The performance of a 'test' transducer constructed to verify both analysis and design theories is also discussed. -3- A CKNOW LEDGEMENTS I wish to express my thanks to my supervisor, Dr.M.Redwood, for his help and guidance throughout the course of this work. This project was carried out under a Ministry of Defence Contract and the test transducer was constructed and tested at the Admiralty Underwater Weapons Establishment. I should also like to acknowledge the advice and assistance afforded by a number of individuals at this Establishment, in particular Dr.D.Stansfield, Mr.G.Bromfield and Mr. D. Evans. Thanks are also due to Lynn Parry who typed this thesis. CO NTENTS Page ABSTRACT 2 ACKNOWLEDGEMENTS 3 CONTENTS 14 LIST OF MAIN SYMBOLS 6 CHAPTER ONE: INTRODUCTION 9 1.1. Aims and Scope of Study 9 1.2. Use of Equivalent Circuits 9 1.3. Analysis 13 1.14. Design Theory 15 1.5. Experimental Verification of Theory 17 CHAPTER TWO: PRELIMINARY CONSIDERATIONS 19 2.].. Piezoelectric Materials for Sonar 19 Transducers 2.2. Electromechanical Analogies 22 2.3. Derivation of the Equivalent Circuit 25 of a Longitudinally Poled Piezo- electric Bar for Parallel Excitation 2.4. Elementary Theory of Conventional 29 Composite Transducers 2.5. Construction of the Ceramic Stack 37 CHAPTER THREE: EQUIVALENT CIRCUITS 140 3.1. Mechanical Elements 42 3.2. Equivalent Circuit of a Tubular Ceramic 45 Segment 3.3. Equivalent Circuit of the Ceramic Stack 50 3.14. Effect of Bonds on the Equivalent 58 Circuit of a Ceramic Stack 3.5. Lateral Effects and Losses 614 3.6. Radiation Impedance of a Single 66 Piston Source 3.7. Average Radiation Impedance of Piston 71 Sources in Regular Plane Arrays CHAPTER FOUR: DESIGN OF PIEZOELECTRIC SONAR 8]. TRANSDUCERS 4.1. Generalized Equivalent Circuit of a 81 Piezoelectric Sonar Transducer -5- Page 4.2. Properties of the Generalized 85 Equivalent Circuit 4.3. Canonic Bandpasa Circuits 89 4.4. Coupled Resonator Bandpass Circuits 95 4.5. Properties of the Alternative 103 Broadband Circuits 4.6. Transducer Design in Lead Zirconate 111 Titanate Type Ceramic CHAPTER FIVE: ANALYSIS 123 5.1. Formulation of the Complete 123 Equivalent Circuit 5.2. Computation of the Frequency 130 Characteristics 5.3. Evaluation of the Time Responses 135 5.4. Analysis of Ladder Networks 147 CHAPTER SIX: EXPERIMENTAL RESULTS AND 150 CONCLU SI ON S 6.1. Characteristics of the Test 150 Transducer 6.2. Comparison of the Measured and 159 Computed Characteristics 6.3. Summary and Conclusions 162 APPENDIX I: Radiated Power and Bandwidth of 166 Shunt Tuned Elementary Transducer APPENDIX II: Program for the Determination 171 of the Average Radiation Impedance in a Regular Array APPENDIX III: Program for the Analysis of a 173 Piezoelectric Sonar Transducer APPENDIX IV: Program for the Analysis of 178 Ladder Networks REFERENCES 183 -6- LIST OF MAIN SYMBOLS a Radius of radiating face A Cross-sectional area normal to the direction of propagation of longitudinal waves B Inverse of the bandwidth s c Velocity of longitudinal waves Ce c(l-k3); effective velocity in a ceramic stack C Generic symbol for electrical capacitance and mechanical compliance in the impedance analogy Cb Bond compliance CC Low frequency compliance of a ceramic stack Co Electrical capacitance of a ceramic segment d33 Effective component of the piezoelectric tensor (strain/field at constant stress) D Electric flux density E Electric field f Frequency centre frequency f=0 Cut-off frequency at lower half-power point Cut-off frequency at upper half-power point Frequency of resonance F Complex representation of force g33 Effective component of the piezoelectric tensor (electric field/stress at constant D) G Electrical conductance 1 Instantaneous value of current I Complex representation of current I k 2w/A; wave number -7- E T k 33 = d 33 /(S 33 . £33) k Effective electromechanical coupling factor e ff Coupling factors associated with filter circuits K = keff/Ci - k ff ); measure of effective coupling K Low frequency value of K 0 L Gqneric symbol for electrical inductance and mass in the impedance analogy L Mass of the radiating head M correction factor for bonding p Number of segments in a ceramic stack P Power radiated or absorbed from the supply by a transducer Maximum power that can be delivered from a given supply (see equation ('4.2)) q Quality factors associated with filter circuits Output or mechanical Q of a transducer r Terminating resistance of normalized filter circuits R Generic symbol for electrical and mechanical resistance and in particular radiation resistance R Generator resistance g R Radiation resistance r 8 Laplace transform variable S33D Effective component of the elastic compliance tensor at constant D - effective component of the elastic 33 - compliance tensor at constant E t Time T Stress U Instantaneous velocity v Instantaneous voltage -8- V Complex representation of voltage V Supply voltage g x: 2 ak x Length in the direction of propagation of longitudinal waves X Radiation reactance Y Generic symbol for admittance Y Young's modulus Z Generic symbol for electrical and mechanical impedance Z0 pAc; mechanical characteristic impedance Zr Radiation impedance fractional bandwidth Dirac delta function T £33 Free dielectric constant A Wavelength of longitudinal waves Ii Function of coupling (see equation ('.'4O)) Particle displacement p Density Electromechanical transformation ratio x Packing factor of a transducer array w = 2irf; angular frequency corresponding frequency (see above) tIo W etc. (I, Actual centre frequency of a normalized filter network -9- CHAPTER I INTRODUCTION 1.1. Aims and Scope of Study The objectives of this study are twofold. First the development of a general technique of analysis applicable to piezoelectric sonar transducers, based on electrical equivalent circuits which accurately represent t1 transducer in the sonic range. Second, the investigation of the possibility of extending the bandwidth of piezoelectric sonar transducers, by a systematic design technique based on equivalent circuits. The scope of the study is restricted to the commonly used extensional type transducers, which employ longitudinal mode operation of the main constituent mechanical elements. 1.2. Use of Equivalent Circuits The analysis of complex electromechanical systems, by means of the direct solution of the intrinsic differential equations, is fraught with difficulties. The preferred and well-established method of attack is to formulate the related 'steady-state' or frequency domain problem in terms of an analogous electrical circuit. The latter problem is generally much more tractable on account of the powerful and well-developed techniques of electrical circuit theory. The recently developed method of modern systems theory, employing the state-space formulation, is advantageous in that it finds the time domain solution directly, while -1 - simultaneously tracing all the variables. At present, however, its scope is severely limited in comparison with the equivalent circuit technique. A further advantage of the equivalent circuit method is that once the basic analogies are set up, it is usually much easier to form the equivalent circuit than to derive the intrinsic system equations. Thus in sonar transducer design and analysis in general and in this study in particular, the equivalent circuit method is used. Where required, the time domain solutions are obtained from the steady state responses by inverse Fourier transformation. In the
Details
-
File Typepdf
-
Upload Time-
-
Content LanguagesEnglish
-
Upload UserAnonymous/Not logged-in
-
File Pages186 Page
-
File Size-