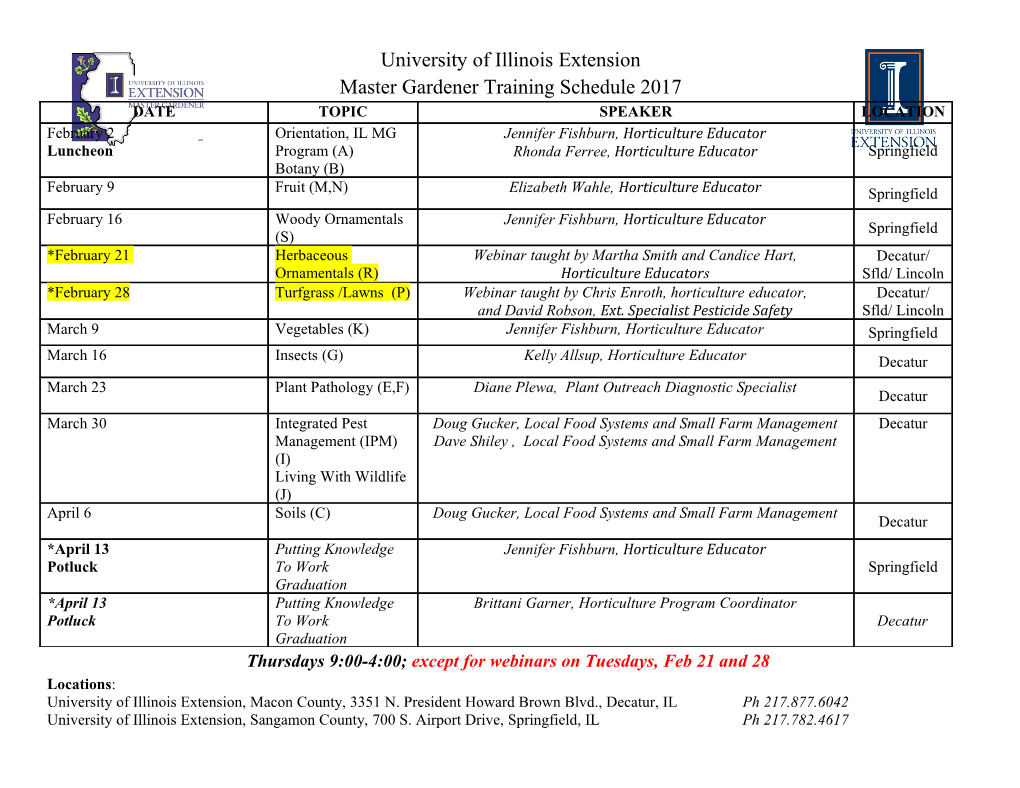
Radio-Frequency Interference (RFI) From Extra-High- Voltage (EHV) Transmission Lines Patrick C. Crane 22 March 2010 1. Introduction The subject of radio-frequency interference (RFI) generated by high-voltage transmission lines has long been of both academic and commercial interest because of concerns about static on AM radio. Today audible noise is of greater concern because many states, counties, and municipalities have noise ordinances and transmission lines are designed to reduce audible noise, while people now expect static on AM radio (Chartier 2009). The subject is of interest today because of the proliferation around the world of low-frequency radio telescopes – including the Low Frequency Array (LOFAR), the Giant Metrewave Radio Telescope (GMRT), the Murchison Widefield Array (MWA), and the Long Wavelength Array (LWA), which is particular interest to the author. The present discussion will concern high-voltage alternating-current (HVAC) transmission lines; high-voltage direct-current (HVDC) transmission lines will be addressed in Appendix A. As described by Pakala and Chartier (1971) power lines are divided into two classes with respect to radio noise: (1) lines with voltages below 70 kV and (2) lines with voltages above 110 kV. Lines in the first class in general exhibit only gap-type discharges and lines in the second class principally exhibit corona discharge. Gap-type discharges are essentially a maintenance issue; corona discharges are a design issue. Extra-high-voltage (EHV) transmission lines have operating voltages of 345 kV or greater. The possibility of interference from such a transmission line first impinged significantly upon radio astronomy (in the United States, at least) in 1984 when the El Paso Electric Company proposed building a 345-kV transmission line from Red Hill, NM to Deming, NM which would run east along US60 to its junction with NM78 (now NM52) and then south along NM78. This route would have crossed both the north and east arms about two miles from the center of the Very Large Array (VLA) radio telescope. In response, the Bureau of Land Management (BLM) hired Vernon L. Chartier of the Bonneville Power Administration (BPA) as a consultant to study the problem and recommend minimum separation distances for the VLA and for the Very Long Baseline Array (VLBA) radio telescope, construction of which began in 1985. Mr. Chartier was well qualified for the task as the Chief High Voltage Phenomena Engineer of BPA’s Division of Laboratories with many years of research in the field. He is still active and when I contacted him ([email protected]), he provided more recent references. Among them is the EPRI Red Book (2008) which is published by the Electric Power Research Institute, Inc. (EPRI); Mr. Chartier sent me the small number of relevant pages because the Red Book sells for $5000. The EPRI is an independent nonprofit 1 organization that conducts research and development relating to the generation, delivery and use of electricity for the benefit of the public. The Red Book (2008) recognizes the BPA method because The most complete empirical method for predicting EMI above 30 MHz is the method developed by the Bonneville Power Administration (Chartier 1983). The BPA method was first developed to predict TVI from overhead lines during rain, but the method has been expanded so that EMI above 30 MHz can be calculated at any frequency, at any distance from the line, at any antenna height, for any bandwidth, and for any detector. Furthermore, it is “the one method that is useful for EMI predictions between 30 and 1000 MHz” and “the most complete empirical method for predicting EMI above 30 MHz.” The BPA model also has been recognized by the International Council on Large Electric Systems (CIGRE, 1996), which is one of the leading worldwide organizations on electric power systems. 2. What Is Corona? Hubbell Power Systems, Inc. (2004) has produced a clear and concise report on corona with the title “What Is Corona?” which is available on the World Wide Web. This report defines corona in the following terms: Corona is a luminous discharge due to ionization of the air surrounding a conductor around which exists a voltage gradient exceeding a certain critical value, based upon IEEE Std 539-1990 (IEEE Corona and Field Effects Subcommittee, 1991) as applied to transmission-line conductors. Or in more physical terms: The corona discharges observed at the surface of a conductor are due to the formation of electron avalanches which occur when the intensity of the electric field at the surface of a conductor exceeds a certain critical value. Furthermore, Any defect on the conductor[,] which projects however slightly above the nominal conductor surface, increases the field intensity in its immediate vicinity...Water drops on the conductor surface provide a multiplicity of projections from which corona discharges can originate...A weathered ACSR conductor generally has a multiplicity of tiny surface defects which project above the nominal surface of the conductor. The presence of corona manifests itself primarily in three ways: 2 1. “Visual corona” – violet-colored light coming from the regions of electrical overstress. Daytime corona cameras and ultraviolet cameras both are used to inspect transmission lines for corona. 2. “Audible corona” – a hissing or frying sound when corona is present. 3. Radio noise – apparently this is the most serious manifestation from the point-of-view of the power company (for radio astronomers, too) because its effects have the longest range. The phenomena associated with corona have been described quantitatively by Chartier (1983): radio noise (RI) over the frequency range 0.1-20 MHz (i.e., AM radio, ham radio); television interference (TVI) over the frequency range 10-1000 MHz, which includes VHF and UHF television and FM radio and, coincidentally, is measured in the radio-astronomy allocation 73.0-74.6 MHz because of the absence of interference; audible noise (AN); and ozone (concentration C). The total power loss per meter of conductor is identified as the corona losses (CL). The presence of more surface defects produces higher levels of corona and related phenomena. Indulkar (2004) discusses the effects of such surface irregularities on the threshold voltage for the onset of corona. He reports that this voltage is directly proportional to an irregularity factor, m0 (0<m0≤1): 1 for smooth, polished, solid, cylindrical conductors; 0.93-0.98 for weathered, solid, cylindrical conductors; 0.87-0.90 for weathered conductors with more than seven strands; and 0.80-0.87 for weathered conductors with up to seven strands. Finally, the Introduction states that corona from EHV transmission lines is primarily a design problem. While that is true – from the point-of-view of a radio astronomer, corona can be a maintenance issue because of such problems as degraded or damaged insulators; contamination with coastal salt, industrial vapors and dust, cement dust, road salt, tire dust and vehicle emissions; agricultural dusts and fertilizers; improper design and/or installation; and loose hardware. 3. Television Interference (TVI) As stated above the method developed by the Bonneville Power Administration (BPA) is the most complete empirical method for predicting radio-frequency interference (RFI) above 30 MHz. The BPA calculation of TVI/phase for a CISPR quasi-peak detector from corona discharge is given by Equation 9.6-4 in EPRI (2008): TVI/phase = 10 + TVIV(E) + TVIS(d) + TVIf + TVIA+ TVID(L), (1) where 3 TVI/phase = electric field (dBμV/m), measured during steady rain with an IEC/CISPRQuasi-Peak (QP) detector with a 1-ms charge time constant, a 550-ms discharge time constant, and 6-dB bandwidth of 120 kHz, for a single phase, E = conductor surface voltage gradient (kV/cm), d = subconductor diameter (cm), f = frequency (MHz), A = altitude above sea level (km), L = distance between antenna and phase (m). Individual terms will be discussed in the following sections. 3.1. Conductor Surface Voltage Gradient TVIV(E) = 120*log(E/16.3), (2) where E = conductor surface voltage gradient (kV/cm). The conductor surface voltage gradient is the single most important factor in determining the corona performance of a high-voltage transmission line. This is indicated by the multiplicative factor of 120 in front of the appropriate (second) term in Equation (1); the multiplicative factors for other terms are 40, 20, and 1. If E changes by a factor of ±2, the TVI will change by ±36 dB. It is so important, also, because its value is entirely a design issue. Therefore, the calculation of its value has long and widely been the subject of investigation. A survey of methods used for such calculations (as of 1979) was published by the IEEE Corona and Field Effects Subcommittee (1979). Seventeen techniques were applied to multiple extra-high-voltage (EHV, ≥345 kV) transmission-line configurations. Empirical techniques were found to agree with exact solutions to within a few percent, so that when applied in the field the overall uncertainty was less than ten percent. A further uncertainty may arise from the definition of the conductor surface voltage gradient; the three definitions used in the survey include the average bundle gradient (A), the average maximum bundle gradient (AM), and the maximum bundle gradient (MB). As a practical matter, Table I of the paper provides parameters for EHV transmission lines with operating voltages of 345 kV, 500 kV, 765 kV, 1100 kV, and 2000 kV (AC) and ±375 kV and ±1000 kV (DC). The computed gradients are listed in Table II. Participant No. 4 is Vernon L. Chartier and he reports only the results of an AM calculation. By 1979, such calculations had migrated to the digital computer and had expanded in capability and complexity. One can see an earlier version of the BPA calculation, suitable for manual calculation, in Pakala and Taylor (1968).
Details
-
File Typepdf
-
Upload Time-
-
Content LanguagesEnglish
-
Upload UserAnonymous/Not logged-in
-
File Pages34 Page
-
File Size-