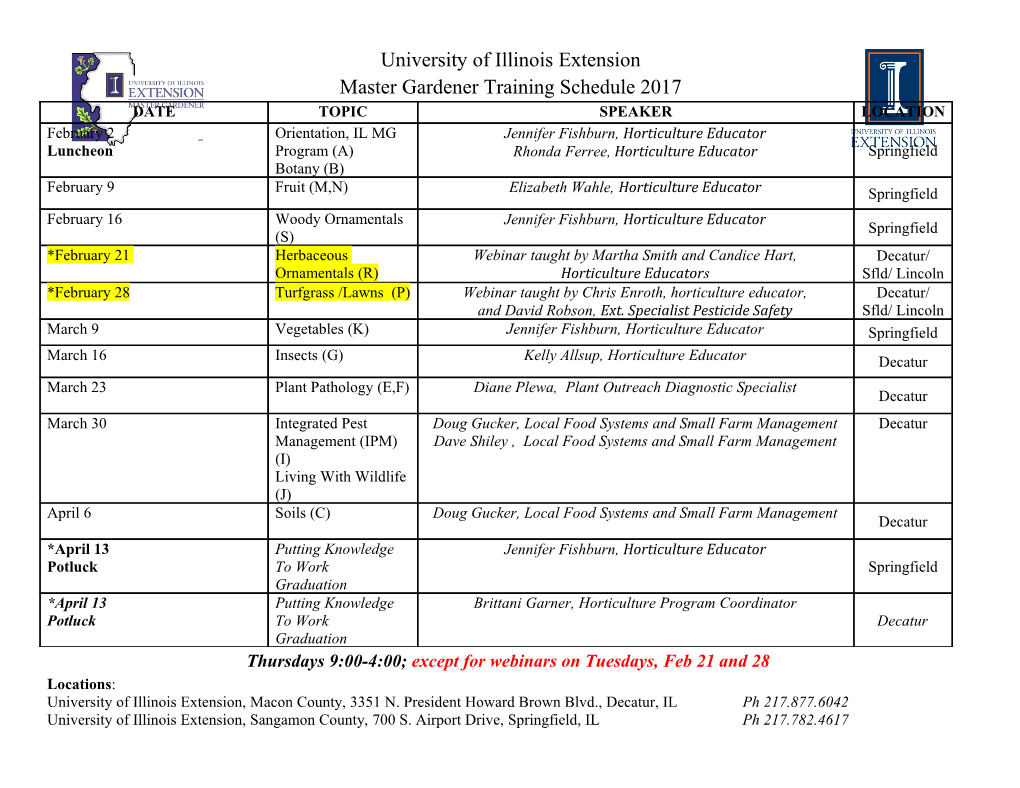
Department of Experimental Physics http://physics.ttk.pte.hu Prospects of THz Pulse Generation with mJ- Leve l Energy and 100 MV/cm Elec tr ic Fie ld J. A. Fülöp1,,,2,*, Z. Ollmann3, L. Pálfalvi3, G. Almási1,3, J. Hebling 1,3 1High-Field THz Research Group, HAS, Hungary 2Attosecond Light Pulse Source of the Extreme Light Infrastructure, Hungary 3Department of Experimental Physics, University of Pécs, Hungary *e-mail: [email protected] .pte .hu Workshop on THz Sources for Time Resolved Studies of Matter, ANL, Argonne, July 30-31, 2012 Applications of High-Energy THz Pulses 1,0 E 1,0 max 0,8 0,6 ) mplitude (a. u.) .. 0,5 aa 0,4 0,2 Spectral Spectral 0,0 0123 Frequency (THz) ric field (a. u ric field tt 000,0 Elec -0,5 -2 0 2 4 6 8 time (ps) Linear THz spectroscopy (Emax ≈ 100 V/cm → 10 fJ pulse energy) graphene, nanotubes, molecular magnets, etc. NliNonlinear THz spec troscopy (Emax ≈ 100 kV/cm → µJlJ pulse energy) THz pump – THz probe measurements of dynamics (Hoffmann et al., PRB, 2009) Manipulation and acceleration of charged particles (Emax ≈ 100 MV/cm → 10 mJ pulse energy) VUV–XUV pulse generation by Thomson-scattering, X-ray free electron laser, … Optical Rectification Conversion 2 2 2 2 2ω d L I −α L 2 sinh [α L 4] efficiency THz eff THz THz ηTHz = 2 3 ⋅e ⋅ 2 depends on: ε 0nv nTHzc [αTHz L 4] THz frequency: 2 ηTHz ∝ ωTHz Material parameters → FOM gr ph Phase matching → velocity matching: vvis = vTHz Figure of merit (FOM): 2 2 2 2 2 2ω deff L I deff L αTHzL << 1 ηTHz = 2 3 FOM NA = 2 ε 0nv nTHzc nv nTHz 2 2 2 8ω deff I 4deff αTHzL >> 1 ηTHz = 2 2 3 FOM A = 2 2 ε 0nv nTHzαTHzc nv nTHzαTHz Materials for Optical Rectification gr d gr αTHz FOM* Material eff n n1.55μm (/V)(pm/V) 800nm nTHTHz (cm-1) (pm2cm2/V2) CdTe 81.8 3.24 2.81 4.8 11.0 GaAs 65.6 4.18 3.59 3.56 0.5 4.21 GPGaP 24. 8 3673.67 3343.34 3163.16 020.2 0720.72 ZnTe 68.5 3.13 3.17 2.81 1.3 7.27 GaSe 28.0 3.13 3.27 2.82 0.5 1.18 168 2.25 4.96 2.18 17 18.2 sLiNbO3 sLN 100K 4.8 4886.6 DAST 615 3.39 2.58 2.25 50 41.5 gr ph gr Velocity matching condition: vNIR = vTHz ⇒ nNIR = nTHz * for L = 2 mm Tilted-Pulse-Front Pumping (TPFP) gr ph vvis ⋅ cosγ =vTHz Hebling et al., ~100 fs typical Opt. Express, 2002 Tilted-Pulse-Front Pumping vs. Line Focusing Stepanov et al., Opt. Express, 2005 Hoffmann & Fülöp, J. Phys. D, 2011 THz Pulse Generation by Optical Rectification in LiNbO3 Using Tilted Pulse Front Pumping Large nonlinear coefficient (deff = 168 pm/V) Velocity matching by tilting the pump pulse front gr ph Hebling et al., Opt. Ex press, 2002 vvis ⋅ cosγ =vTHz Highest THz pulse energy from a table-top source 0. 25 μJ Stepanov et al., Opt. Express , 2005 10 μJ Yeh et al., Appl. Phys. Lett., 2007 50 μJ Stepanov et al., Appl. Phys. B, 2010 125 μJ Fülöp etlt al., Op t. L e tt., 2012 Further increase of THz energy is in sight – optima l conditions (pump pulse duration & wavelength, crystal temperature & length) Fülöp et al., Opt. Express, 2011 – by increasing the pumped area in optimized setups Pálfalvi et al., Appl. Phys. Lett., 2008 Fülöp et al., Opt. Express, 2010 Effective Interaction Length Pump propagation distance, ζ [mm] -20 -10 0 10 20 Pulse front tilt: s] pp [ (a) n dε τ 2 tanγ = − λ ng dλ uration, uration, dd 2L d 1 angular material ump pulse ump pulse PP LiNbO3 dispersion dispersion λp = 800 nm 2 0 Fp = 5.1 mJ/cm τ = 50 fs 0 GVD parameter: Ωpm = 1 THz %] [[ τ = 350 fs 0 4 τ = 600 fs 0 −1 2 2 d(vg ) λ ⎡ ⎛ dε ⎞ d n⎤ D = = ⎢n⎜ ⎟ − 2 ⎥ efficiency efficiency nn (b) L d λ c ⎣ ⎝ d λ ⎠ d λ ⎦ 2 eff z generatio z HH Martínez et al., JOSA A , 1984 T 0 -5 0 5 Hebling, Opt. Quantum Electron., 1996 THz propagation distance, z [mm] Fülöp et al., Opt. Express, 2010 Effective Interaction Length Limiting effect Possible solution Use longer Fourier-limited pump pulses Change of pump pulse duration inside the medium Use materials requiring smaller pulse- front tilt Absorption at THz frequencies Cool the crystal to reduce α Absorption coefficient α Use longer pump wavelength to Multi-photon absorption (MPA) of the suppress low-order MPA pump Use materials requiring smaller pulse- front tilt Walk-off Use large pump beam (and energy): → Optimized imiggganig system → Contatc grating (no imaging) Optimization of the Pump Pulse Length Fülöp et al., Opt. Express, 2011 3.0 LiNLiNbObO3 300 K L ≤ 10 mm 2.5 2.8 MV/cm 100 K 2 Ip, max = 40 GW/cm 10 K MV/cm] MV/cm] λ = 1064 nm [[ [[ p 2.0 2.3 MV/cm ic field ic field rr rr 151.5 absorption coefficient of 1.0 LiNbO3: ak elect ak elect ee ee p p T [K] α [1/cm] 1.0 MV/cm 0.5 10 0.35 240 kV/cm 0.0 100 2.1 0 500 1000 1500 300 16 pump pulse duration [fs] experimental value: 110 kV/cm Hoffmann et. al., Phys. Rev. B., 2009 Optimization of the Pump Pulse Length 25 300 K 300 K 100 K 100 K 10 K 20 10 K 10 15 [%] y [mJ] yy 10 5 efficienc 2.0% THz energ 5 0 0 0 500 1000 1500 0 500 1000 1500 ppppump pulse duration [][fs] ppppump pulse duration [][fs] τp = 500 fs THz energy ≈ 25 mJ Ep = 200 mJ THz field = 2.8 MV/cm (unfocused) 2 Ip, max = 40 GW/cm → 10 MV/cm level using imaging → 100 MV/cm level using focusing Optimization of the Pump Pulse Length Frequency at the spectral peak 1,5 LiNbO3 L ≤ 10 mm 300 K 2 Ip, max = 40 GW/cm 100 K lp = 1064 nm [THz] 10 K 00 1,0 Ω Phase matching ency, adjusted to the uu spectral peak 0,5 eak freq pp 0 200 400 600 800 1000 pump pulse duration [fs] New Appilcation Possibilities for THz Pulses with High Electric Field THz beam Acceleration Plettner et al., Phys. Rev. Spec. Top. – Accel. and Beams 9, 111301 (2006) Beam deflection, focusing Ple ttner etlt al., Phys. Rev. Spec. Top. – AlAccel. andBd Beams 12, 101302 (2009) 1 GV/m = 10 MV/cm peak field strength is needed New Appilcation Possibilities for THz Pulses with High Electric Field Enhancement of HHG E. Balogh et al., PRB, 2011 K. Kovács et al., PRL, 2012 Longitudinal compression of electron bunches → single-cycle MIR…XUV pulse generation Hebling et al., arXiv:1109.6852 Hebling et al., presentation on Monday Electron undulation HbliHebling etlt al., arXiv:1109 . 6852 Proton acceleration (40 → 100 MeV requires 30 mJ THz energy) → hadron therapy Pálfalvi et al., in preparation Towards mJ Level THz Pulses: Experiment 1000 experiment In collaboration with 1.6 power fit S. Klingebiel, F. Krausz, S. calculation square law Karsch J] 100 μ MPQ, Garching [ yy Further possibilities: 10 Hz energ • Optimal pump duration TT T =300 K • Cooling the LiNbO lp = 1030 nm 3 • Contact grating tp = 1.3 ps (not Fourier-limited) 1 1 10 100 pump pulse energy [mJ] Comparison with earlier results: Stepanov et. al., Appl. Phys. B, 2010: Fülöp et al., Opt. Lett. 2012: ETHz = 50 µJ ×2.5 125 µJ η = 0.05% ×5 0.25% THz Beam Profiles LN x [m Contact grating crystal m] THz 4 2 No limit for lateral 0 1.0 -2 (a) size ()(c) -4 pump (b) No imaging optics (c) [arb.[arb. u.] u.] [arb. u.] yyyy Pálfa lv i e t a l., APL, 2008 yy 050.5 Ollmann et al., APB, LN THz crystal submitted pump HzHz intensit intensit Hz intensit TTTT TT Non-optimized 0.0 (a) 8 4 0 -4 -8 x [mm] Optimized THz x [m THz generation m] efficiency [arb. u.] 2 4 Grating image is 1E-3 2 pump 8E-4 tangential to ]] mmmm 0 pulse front 0 6E-4 [m [m -2 4E-4 ξξ Longer focal -4 2E-4 -2 0 length lens LN crystal Fülöp et al., (b) -4 -2 0 2 4 Opt. Express, 2010 ζ [mm] Optimizing the TPFP Setup Containing Imaging LiNbO3, λp = 800 nm Fülöp et al., Opt. Express , 2010 90 θi optimal angle of incidence θLittrow θ +10 60 Littrow o θLittrow-10 cidence nn 30 angle of i of angle 1800 1/mm 2200 1/mm 0 1200 1600 2000 2400 1/d (1/mm) 1 MV/cm field and 0.1% efficiency reached by applying our above results Hirori et al., Appl. Phys. Lett., 2011 Design of Contact Grating on LiNbO3 Contact grating for THz generation proposed: Pálfalvi et al., APL, 2008 Index matching by glass: Nagashima et al., Japan J. Appl. Phys., 2010 Re frac tive in dex matc hing liqu id (RIML): Ollmann e t a l., APB, su bm itte d Diffraction efficiency for order -1 RIML for BK7, d = 350 nm pitch grating THz Energy vs. Pump Energy 105 104 LN (tilted pulse front) 103 J) 102 rgy (n 1 LN (()Yeh, 2008) ee 10 LN (Yeh, 2007) 100 LN (Stepanov, 2005) lse en LN ((pStepanov, 2008) uu -1 10 LN (Fülöp, 2012) ZnTe (Blanchard, 2007) 10-2 THz p ZnTe ((,)Löffler, 2005) -3 10 ZnTe (collinear) 10-4 10 100 1000 10000 100000 pump pulse energy (μJ) Reconsidering Semiconductors for THz Generation Materi- λp = 800 nm λp = 1064 nm λp = 1560 nm al MPA γ MPA γ MPA γ MPA: lowest-order ZnTe 2PA collinear 2PA 22.4° 3PA 28.6° multi-photon pump absorption GaSe 2PA 16.5° 2PA 25.9° 3PA 30.2° γ : pulse-front tilt angle LiNbO3 3PA 62.7° 4PA 63.4° 5PA 63.8° Ω = 1THz1 THz Fülöp et al., 101 0 Opt.
Details
-
File Typepdf
-
Upload Time-
-
Content LanguagesEnglish
-
Upload UserAnonymous/Not logged-in
-
File Pages22 Page
-
File Size-