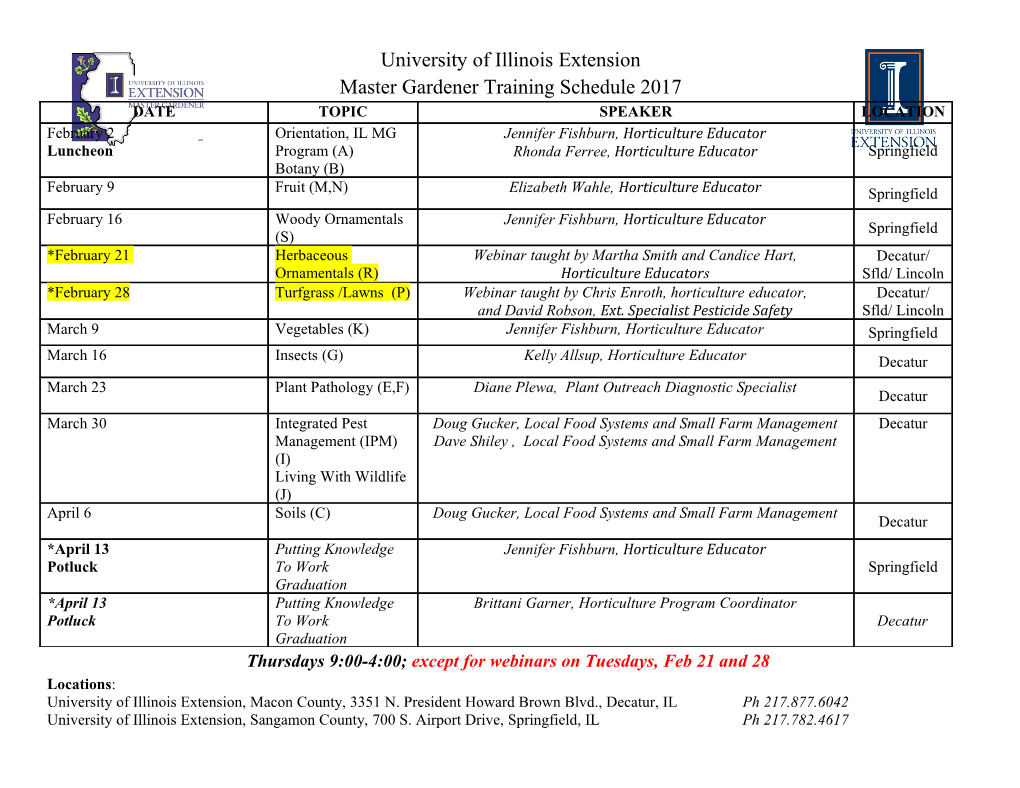
Conclusion Freshwater environments contain a diverse array of terrestrial organisms which have adapted to life underwater. In making the transition from an environment with high oxygen availability to one where oxygen availability is low, many of these terrestrial invaders continue to rely on the atmosphere as their respiratory medium, using adaptations as varied as the organisms themselves. This thesis is broadly concerned with the mechanisms evolved by two dissimilar groups of organisms, insects and plants, to solve their common problem of obtaining oxygen while underwater. The insect’s air-filled tracheal system is surprisingly well suited to functioning in the aquatic environment. Gas exchange in this system is diffusive: oxygen from the atmosphere diffuses into the tracheal system through spiracles, while carbon dioxide diffuses out. Insects need only cover their spiracles with an air bubble to breathe underwater. But the properties of an air bubble (i.e., the solubility of its gases and buoyancy) confer benefits besides acting as a simple oxygen reserve. Comparison of two aquatic bugs, backswimmers Anisops deanei and water boatmen Agraptocorixa eurynome, demonstrates the diverse ways in which aquatic insects use air bubbles while submerged. Backswimmers use a small bubble of air as a self-contained buoyancy and respiratory apparatus. Hairs fringing the abdominal air-store form a dense barrier over the surface of the bubble, greatly reducing the area available for diffusion. In concert with large haemoglobin cells, this allows the backswimmer to stabilise the oxygen partial pressure and buoyancy of its air-store. In contrast, the air bubble carried by water boatmen has a high degree of contact with the surrounding water, covering the entire ventral surface of the insect’s flattened body. Water boatmen use their bubble as a gas gill, fanning water over its surface with their oar-like hind legs. By doing so, they extract sufficient amounts of dissolved oxygen from the surrounding water to satisfy their respiration. Obtaining this oxygen comes at a cost, as a thin boundary layer necessary for oxygen diffusion into the gill also promotes the diffusion of nitrogen out. In the past, technical limitations have prevented direct measurement of oxygen partial pressures within air bubbles. Previous studies relied on indirect measurements of dissolved oxygen in sealed chambers containing a submerged aquatic insect. In this thesis, measurements made with new fibre-optic oxygen probes have shown oxygen fluctuations occurring within the air-stores of submerged insects in real time. Combined with measurements of buoyancy, this has produced the first empirical 114 measurements of the behaviour of a functioning gas gill. Data from oxygen probes have also been used to determine the oxygen-binding properties of backswimmer haemoglobin in situ, thus avoiding the problems associated with changes in affinity due to altered conditions in vitro. While aquatic insects rely on oxygen carried from the surface or diffusive uptake from the surrounding water to supply their oxygen requirements, many aquatic plants generate convective flows of air to ventilate their submerged rhizomes. Convective ventilation through a submerged rhizome requires that the plant have an influx point and an efflux point connected by a continuous air-filled cavity, and a means of generating a pressure gradient across them. While most aquatic plants ventilate a simple interconnecting cavity, the gas canal system of the sacred lotus (Nelumbo nucifera) displays a degree of specialisation which is truly remarkable. Gas flow within all other emergent aquatic plants occurs as a result of pressurised air from young leaves flowing into a common interconnecting lacunal system and venting though old or damaged leaves. The occurrence of vent points is therefore haphazard, with convective air flow only occurring through those lengths of the rhizome between an influx and an efflux point. In contrast, the gas canal system of the lotus is highly ordered, with multiple independent canal pairs channelling gas from pressurising leaf lamina to specialised efflux points. Multiple independent gas canal pairs allow the lotus to generate flow in opposite directions within the same length of rhizome or petiole. This provides an important benefit to the creeping rhizome of the lotus, because it ensures that ventilation occurs right to the growing tip of a shoot as it extends into anoxic sediment. Aquatic plants with a single interconnecting cavity must rely on oxygen diffusion from the nearest ventilated section of rhizome to supply their growing shoots, potentially placing a constraint on the length of an extending shoot. The lotus also possesses a specialised vent organ in the centre of every leaf, where the apertures of large stomata form a conduit between the atmosphere and the gas canals of the leaf and petiole. Uniquely, the action of these stomata regulate the direction of convective gas flow. The intricate arrangement of gas canals and the obvious diurnal control of the central plate stomata suggest that these features in the lotus exist to perform a specific function. If ventilation of gas canals provided the only benefit to the plant, then it could be achieved with a much simpler arrangement of canals and permanently open efflux points. The apparently contradictory function of the central plate stomata in short circuiting two-thirds of the pressurising leaf blade is also puzzling. A potential 115 explanation lies in the benefits to be gained from channelling readily available carbon dioxide from the sediment into the leaf blade. While previous studies on aquatic macrophytes have shown limited use of sediment-derived carbon, none of the plants examined possessed a gas canal system comparable with that of the lotus. A focused examination of daily carbon dioxide fluxes within the gas canals, coupled with isotope tracer experiments, would yield the necessary data to test this hypothesis. 116 References Cited Andersen NM, Weir TA (2004) Australian water bugs. Their biology and identification (Hemiptera-Heteroptera, Gerromorpha and Nepomorpha). Collingwood: CSIRO Publishing. 344pp. Arieli R, Lehrer C. (1988). Recording of locust breathing frequency by barometric method exemplified by hypoxic exposure. Journal of Insect Physiology 34, 325-328. Armstrong J, Afreen-Zobayed F, Armstrong W. (1996). Phragmites die-back: Sulphide- and acetic acid-induced bud and root death, lignifications, and blockages within aeration and vascular systems. New Phytologist 134, 601-614. Armstrong J, Armstrong W. (1991). A convective through-flow of gases in Phragmites australis (Cav) Trin Ex Steud. Aquatic Botany 39, 75-88. Armstrong J, Armstrong W, Beckett PM. (1992). Phragmites australis: Venturi- and humidity-induced pressure flows enhance rhizome aeration and rhizosphere oxidation. New Phytologist 120, 197-207. Armstrong W, Armstrong J, Beckett PM. (1996). Pressurised aeration in wetland macrophytes: Some theoretical aspects of humidity-induced convection and thermal transpiration. Folia Geobotanica and Phytotaxonomica 31, 25-36. Bailey PCE. (1987). Abundance and age-specific spatial and temporal distribution in two waterbug species, Anisops deanei (Notonectidae) and Ranatra dispar (Nepidae) in three farm dams in South Australia. Oikos 49, 83-90. Baker GM, Wright EA. (1977). Effects of carbon monoxide on insects. Bulletin of Environmental Contamination and Toxicology 17, 98-104. Bare CO. (1929). Haemoglobin cells and other studies of the genus Buenoa (Hiemiptera, Notonectidae). The University of Kansas Scientific Bulletin 18, 265-349. Bendix M, Tornbjerg T, Brix H. (1994). Internal gas-transport in Typha latifolia L. and Typha angustifolia L. 1. Humidity-induced pressurization and convective throughflow. Aquatic Botany 49, 75-89. Bergtrom G. (1977). Partial characterisation of haemoglobin of the bug, Buenoa confusa. Insect Biochemistry 7, 313-316. Bergtrom G, Gittelman S, Laufer H, Ovitt C. (1976). Haemoglobin synthesis in Buenoa confusa (Hemiptera). Insect Biochemistry 6, 595-600. Blaylock AJ, Seymour RS. (2000). Diaphragmatic nets prevent water invasion of gas canals in Nelumbo nucifera. Aquatic Botany 67, 53-59. Brix H. (1990). Uptake and photosynthetic utilization of sediment-derived carbon by Phragmites australis (Cav) Trin Ex Steudel. Aquatic Botany 38, 377-389. 117 Brix H, Sorrell BK, Orr PT. (1992). Internal pressurization and convective gas flow in some emergent freshwater macrophytes. Limnology and Oceanography 37, 1420- 1433. Buchel HB, Grosse W. (1990). Localization of the porous partition responsible for pressurized gas-transport in Alnus glutinosa (L) Gaertn. Tree Physiology 6, 247-256. Burmester T, Hankeln T. (2007). The respiratory proteins of insects. Journal of Insect Physiology 53, 285-294. Chaui-Berlinck JG, Bicudo JEPW. (1993). Factors affecting oxygen gain in diving insects. Journal of Insect Physiology 40, 617-622. Chaui-Berlinck JG, Bicudo JEPW, Monteiro LHA. (2001). The oxygen gain of diving insects. Respiration Physiology 128, 229-233. Colmer TD. (2003). Long-distance transport of gases in plants: a perspective on internal aeration and radial oxygen loss from roots. Plant, Cell and Environment 26, 17-36. Comstock JH. (1887). Note on the respiration of aquatic bugs. The American Naturalist 21, 577-578. Constable JVH, Longstreth DJ. (1994). Aerenchyma carbon dioxide can be assimilated in Typha latifolia leaves. Plant Physiology 106, 1065-1072. Curran M, James P, Allaway WG. (1996).
Details
-
File Typepdf
-
Upload Time-
-
Content LanguagesEnglish
-
Upload UserAnonymous/Not logged-in
-
File Pages10 Page
-
File Size-