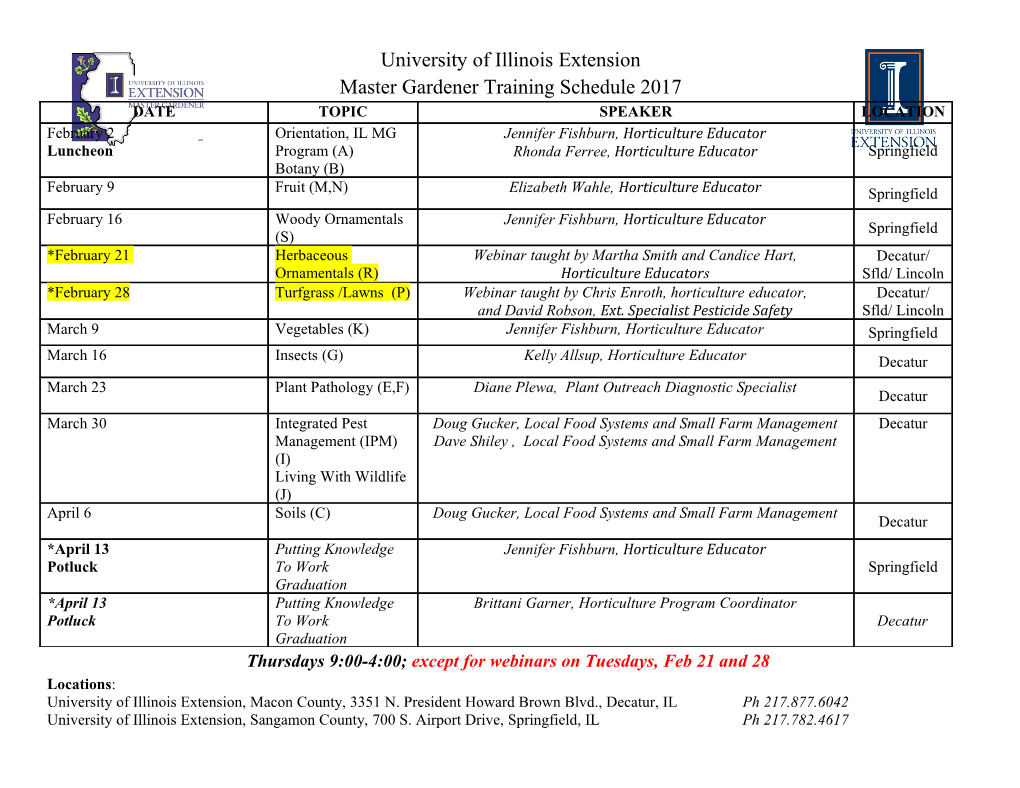
Lecture 18 - Beyond the Standard Model Why is the Standard Model incomplete? • Grand Unification • Baryon and Lepton Number Violation • More Higgs Bosons? • Supersymmetry (SUSY) • Experimental signatures for SUSY • 1 Why is the Standard Model incomplete? The Standard Model does not explain the following: The relationship between different interactions • (strong, electroweak and gravity) The nature of dark matter and dark energy • The matter-antimatter asymmetry of the universe • The existence of three generations of quarks and leptons • Conservation of lepton and baryon number • Neutrino masses and mixing • The pattern of weak quark couplings (CKM matrix) • 2 Grand Unification The strong, electromagnetic and weak couplings αs, e and g are 15 running constants. Can they be unified at MX 10 GeV? ≈ We would also like to include gravity at the Planck scale 19 MX 10 GeV. Is string theory a candidate for this? ≈ 3 SU(5) Grand Unified Theory (GUT) Simplest theory that unifies strong and electroweak interactions (Georgi & Glashow) 15 Introduces 12 gauge bosons X and Y at MX 10 GeV ≈ These are known as leptoquarks. They make charged and neutral couplings between leptons and quarks Explains why Qν Qe = Qu Qd − − Existence of three colors is related to fractional quark charges Predicts proton decay p π0e+ → 4 2 Prediction of sin θW Loop diagram with ff¯ pair couples a Z0 boson to a photon In electroweak theory the Z0 and γ are orthogonal states The sum of loop diagrams over all fermion pairs must be zero: 2 2 P QI3 X Q(I3 Q sin θW )=0 sin θW = 2 − P Q In a Grand Unified Theory (GUT) the sum is taken over a fermion supermultiplet [νe,e,dr,dg,db] 2 2 sin θW = 0.375, but at electroweak scale sin θW = 0.22! 2 GUT predicts the running of sin θW from 0.215 at √s = MW to 0.375 at 1015 GeV 5 Proton Decay The leptoquark couplings in GUTs do not conserve baryon and lepton number, only the combination B-L 4 0 + MX 31 Γ(p π e ) = 0 τp 2 5 10 years → 6 ∝ α mp ≈ This is age of universe - not a problem for our existence ≫ Large underground experiments (Kamiokande ...) have looked for 32 proton decays. None seen so τp > 2 10 years. × Minimal SU(5) GUT is ruled out by absence of proton decay signal Non-minimal SO(10) GUT is not ruled out. Predicts different decay modes, e.g. p K+ν¯. → 6 Matter-Antimatter Asymmetry The Sakharov conditions for generating the matter-antimatter asymmetry of the universe: C and CP violation in quark or lepton decays. • Baryon number violation. • Departure from thermal equilibrium during the early universe. • Baryogenesis: Non-conservation of baryon number in quark decays and CP violation between quark and antiquark decays. Note that CP violation due to CKM matrix is too small by 109 × Leptogenesis: Non-conservation of lepton number and CP violation in the lepton sector. This leads to non-conservation of baryon number by conservation of B-L. Could be associated with neutrino masses and mixing 7 Multiple Higgs Doublets The Standard Model only requires one Higgs doublet, and one physical Higgs boson H0 It is straightforward to increase the number of doublets, e.g. A doublet that couples to d-type quarks and charged leptons A doublet that couples to u-type quarks Suppresses Higgs contributions to flavour-changing neutral currents In a two Higgs doublet model there are eight φ variables of which three get “eaten” during electroweak symmetry breaking. There are two vacuum expectation values v1, v2 with 2 2 2 tan β = v1/v2, and v1 + v2 = v where v = 246 GeV There are five physical Higgs bosons H+, H−, h0, H0, A0 N.B. there are no charged Higgs bosons in the Standard Model! 8 Experimental Signatures for Charged Higgs For a charged Higgs 100 < MH+ < 170 GeV: t bH+ H+ W +h0 h0 b¯b → → → For a light charged Higgs MH+ < 100 GeV: + − + − + − − e e H H H c¯b H τ ντ → → → Indirect constraints from + + rare decays B τ ντ → and b sγ. → Expressed as limits in + MH and tan β. 9 Supersymmetry (SUSY) Every Standard Model (SM) particle gets a supersymmetric (SUSY) partner: New S = 1/2 fermions New S = 0 bosons (sfermions) (neutralinos and charginos) Quarks Squarks Gluon Gluino ⇔ ⇔ Leptons Sleptons Photon Photino ⇔ ⇔ Neutrinos Sneutrinos W,Z bosons Wino,Zino ⇔ ⇔ Higgs boson Higgsino ⇔ 10 Pros and Cons of SUSY Solves mass hierarchy problem (GUT Electroweak scale) ≫ Higgs and fermion loop contributions cancel precisely with SUSY partners at all mass scales between 102 and 1014 GeV Suppresses flavour-changing neutral currents The lightest neutralino is a dark matter candidate No experimental evidence yet for SUSY particles SUSY particle masses and mixings are unknown Many new parameters compared to Standard Model String theories that include gravity are naturally supersymmetric 11 The Minimal Supersymmetric Model (MSSM) The MSSM requires two Higgs doublets: The masses of the lightest Higgs, h0 and H± are 100 GeV ≈ The MSSM conserves R-parity: The number of SUSY particles is conserved (can’t change SUSY particles into normal particles) The MSSM assumes minimal flavour violation (MFV) Flavour couplings of SUSY partners are same as Standard Model All data are consistent with MFV Indirect constraints on the allowed parameters of the MSSM ⇒ 12 SUSY masses from Direct Searches From not seeing anything at LEP-II: chargino masses > 100GeV sleptonmasses > 100 GeV From not seeing anything at the Tevatron: gluino mass > 300 GeV squark masses > 300 GeV ... but a light stop squark is still allowed > 100 GeV From these can infer a lower bound on the lightest SUSY particle neutralino masses > 50GeV (LSP) This still allows the LSP to be the dark matter of the universe! SUSY Higgs masses: 114 < mh < 150 GeV mH+ > 80 GeV mA > 90 GeV 13 Squarks and Gluinos at the Large Hadron Collider An example of a signature for SUSY at the LHC: Cascade from gluino to lightest neutralino (stable) gluino squark(+¯q) neutralino(+q) slepton(+ℓ¯) LSP (+ℓ) → → → → Can reconstruct gluino, squark and neutralino masses from measurements of leptons, quarks and missing energy 14 International Linear Collider (ILC) Reference Design http://www.linearcollider.org February 2007 500 GeV e+e− collider 30 km long Cost $ 6.9B e+e− sleptons The next thing after LHC? → 15.
Details
-
File Typepdf
-
Upload Time-
-
Content LanguagesEnglish
-
Upload UserAnonymous/Not logged-in
-
File Pages15 Page
-
File Size-