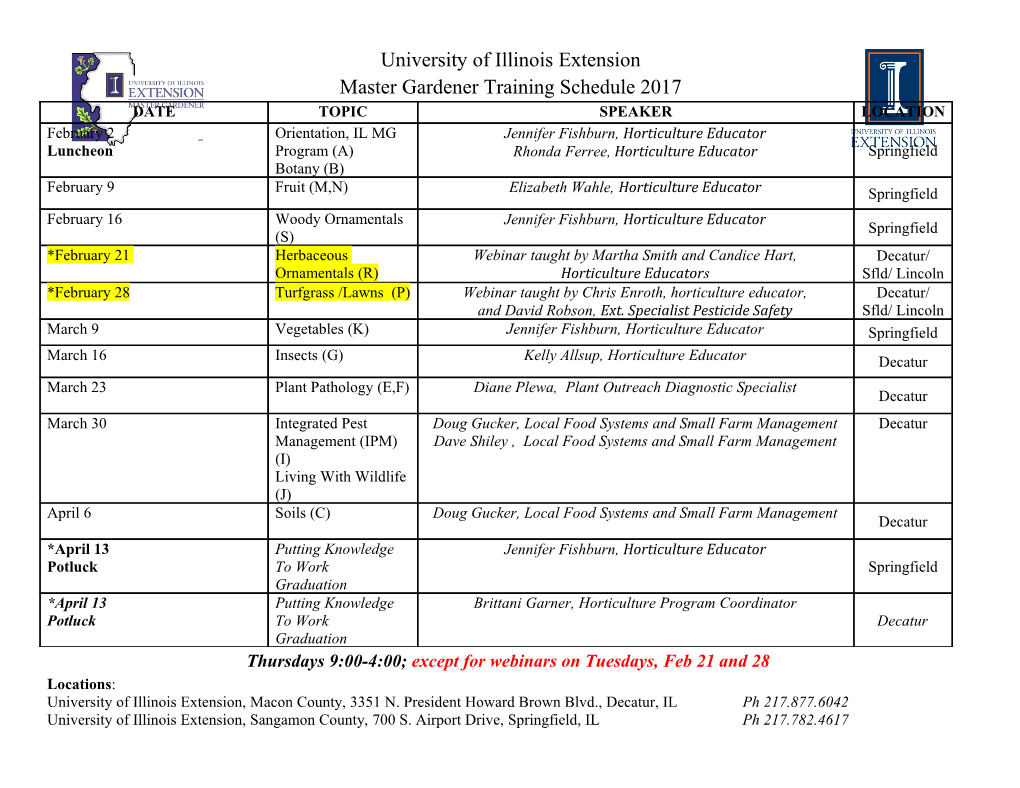
Pure Appl. Geophys. 174 (2017), 925–936 Ó 2016 The Author(s) This article is published with open access at Springerlink.com DOI 10.1007/s00024-016-1432-7 Pure and Applied Geophysics Assessment of Quantitative Aftershock Productivity Potential in Mining-Induced Seismicity 1 1 MARIA KOZłOWSKA and BEATA ORLECKA-SIKORA Abstract—Strong mining-induced earthquakes exhibit various 1. Introduction aftershock patterns. The aftershock productivity is governed by the geomechanical properties of rock in the seismogenic zone, mining- induced stress and coseismic stress changes related to the main Aftershock distribution and productivity depend shock’s magnitude, source geometry and focal mechanism. In order on various factors controlling stress regime in seis- to assess the quantitative aftershock productivity potential in the mogenic layer. The spatial distribution of aftershocks mining environment we apply a forecast model based on natural seismicity properties, namely constant tectonic loading and the correlates with static (e.g. King et al. 1994) as well as Gutenberg-Richter frequency-magnitude distribution. Although transient dynamic stress changes (e.g. Kilb et al. previous studies proved that mining-induced seismicity does not 2000) caused by the main shock. Aftershocks tend to obey the simple power law, here we apply it as an approximation of seismicity distribution to resolve the number of aftershocks, not occur in areas of increased stress; however, they are considering their magnitudes. The model used forecasts the after- also observed within the main shock’s rupture zone, shock productivity based on the background seismicity level where the net stress is believed to drop during an estimated from an average seismic moment released per earthquake earthquake. Such on-fault aftershocks are the result of and static stress changes caused by a main shock. Thus it accounts only for aftershocks directly triggered by coseismic process. In this heterogeneous slip causing heterogeneous coseismic study we use data from three different mines, Mponeng (South stress change on fault’s surface (Helmstetter and Africa), Rudna and Bobrek (Poland), representing different geology, Shaw 2006). The duration and productivity of an exploitation methods and aftershock patterns. Each studied case is treated with individual parameterization adjusted to the data spe- aftershock sequence were proved to depend on the cifics. We propose the modification of the original model, i.e. rock type, its rheological properties and ambient including the non-uniformity of M0, resulting from spatial correlation temperature (Ben-Zion and Lyakhovsky 2006; Yang of mining-induced seismicity with exploitation. The results show that, even when simplified seismicity distribution parameters are and Bez-Zion 2009). Such a large number of factors applied, the modified model predicts the number of aftershocks for controlling the occurrence of aftershocks makes their each analyzed case well and accounts for variations between these modeling challenging—so far it was mostly focused values. Such results are thus another example showing that coseismic on specific aspects of the sequences rather than the processes of mining-induced seismicity reflect features of natural seismicity and that similar models can be applied to study the overall and detailed prediction. The spatial analyses aftershock rate in both the natural and the mining environment. of aftershocks are based mostly on rate-and-state model of Dieterich (1994), which utilizes coseismic Key words: Mining-induced seismicity, aftershocks, static stress transfer. stress changes distribution, or main shock slip models (Das and Henry 2003; Woessner et al. 2006). The time decay of aftershocks is usually considered in terms of Omori-Utsu law (Utsu et al. 1995), which parameters are main shock’s magnitude dependence (Hainzl and Marsan 2008). In order to predict the number of aftershocks directly triggered by the main shock Hainzl et al. Electronic supplementary material The online version of this (2010) formulated the quantitative earthquakes fore- article (doi:10.1007/s00024-016-1432-7) contains supplementary material, which is available to authorized users. cast model based on coseismic static stress changes. According to the authors, the number of aftershocks 1 Institute of Geophysics Polish Academy of Sciences, Ksie˛cia Janusza 64, 01-452 Warsaw, Poland. E-mail: depends on the long-term tectonic loading of the area [email protected] and coseismic stress change, similarly as in a rate- 926 M. Kozłowska, B. Orlecka-Sikora Pure Appl. Geophys. and-state based model. In the model of Hainzl et al. proposed forecast to three mining catalogs. They (2010); however, the long-term tectonic loading is represented different mines, mining methods and constant in time and equals an average seismic rock properties to consider various aftershock pat- moment released by a background seismicity, calcu- terns. To account for spatial correlation of mining- lated analytically based on its frequency-magnitude induced seismicity with exploitation we propose the distribution parameters. non-uniform seismic moment release based on the Induced seismicity studies show that strong background seismicity probability density function induced events, similar to natural ones, can be fol- (PDF). lowed by aftershocks representing various patterns. The procedures applied after strong earthquake in This variability may originate from different many mines, for example in Polish copper mines, geomechanical properties of a rock in a seismogenic aimed at minimizing the aftershocks’ risk, rely zone, differences in the main shock’s rupture type, mainly on tremor’s energy, and thus, do not take into geometry and dynamics, but can also be partly con- account the detailed characteristics of observed seis- trolled by the exploitation parameters. It was already micity. The modeling presented in this study may proven that coseismic stress changes caused by strong contribute to better assessing the aftershock’s risk and mining events can influence the following seismicity contribute in improving the post-earthquake proce- distribution (Orlecka-Sikora 2010; Orlecka-Sikora dures applied in mines. et al. 2012; Kozłowska et al. 2015). Modeling of the seismicity rate, based on Coulomb stress changes and the rate-and-state friction law, can be successfully 2. Model applied to induced seismicity data and help to predict the spatial and temporal distribution of aftershocks of According to Hainzl et al. (2010) the number of strong mining events (Kozłowska et al. 2015). directly triggered aftershocks can be calculated based However, in contrast to natural seismicity, the mining on the long-term average of seismic moment released loading is irregular and changes in time. The time- per earthquake, M0, in the studied seismogenic vol- varying exploitation progress and geometry entails ume. M0 is estimated from the frequency-magnitude transient character of mining-induced seismicity and distribution parameters: b-value, the minimum and a tendency to form clusters correlated in space and maximum magnitude, Mmin and Mmax: time with mining activities (e.g. Gibowicz b 10ð1:5ÀbÞðMmaxÀMminÞ 1 9:1þ1:5Mmin À M0 ¼ 10 ½Nm: 1997, 2006; Orlecka–Sikora and Lasocki 2002; 1:5 À b 1 À 10ÀbðMmaxÀMminÞ Kozłowska 2013). The magnitude distribution of ð1Þ mining seismicity is complex and often multi-modal (e.g. Lasocki 2008). Observed focal mechanisms are Here, the M0 value is assumed constant in space directly coupled to the mining geometry, and thus and long-term seismicity is assumed to follow the vary across the seismogenic zone (e.g. Hasegawa Gutenberg–Richter distribution. The number of trig- et al. 1989), which stands in contrast to uniform focal gered aftershocks Na is calculated by summing K mechanism distributions assumed while estimating values of static stress change, DCFF, each one rep- the average scalar seismic moment release per resenting separate subvolume dV, over the whole earthquake (Catalli et al. 2008). analyzed volume, according to equation: Despite all these unique features, substantially dV XK different from the properties of the tectonic earth- Na DCFF Á H½DCFF; ð2Þ M0 quake process, there is some evidence that models k¼1 tested for natural seismicity give satisfying results where H½DCFF denotes the Heaviside function: also when applied to the mining environment (e.g. H½¼DCFF 1 for DCFF C 0 and 0 elsewhere. The Kozłowska et al. 2015). To test the Hainzl et al. stress change is expressed in terms of a Coulomb (2010) model and to study the aftershock productivity failure function, DCFF = Ds ? l•(Dr ? Dp), potential in mining-induced seismicity we applied the where s is the shear stress in the slip direction, r is Vol. 174, (2017) Aftershock Productivity Potential in Mining Seismicity 927 the normal stress, l is the coefficient of friction and 25,000 events during the following 7 days. Detection p is the pore pressure. The positive change of static and location of a part of these events was made stress moves the fault towards failure, and thus possible by an acoustic sensors’ network installed in increases the probability of aftershock. Mponeng mine within the JAGUARS project (Ja- In our study we calculate DCFF using Coulomb panese-German Underground Acoustic Emission 3.0 software (Lin and Stein 2004; Toda et al. 2005), Research in South Africa) held in 2007–2009. The assuming zero pore pressure. The software requires network was capable of recording seismic events with rectangular source geometry
Details
-
File Typepdf
-
Upload Time-
-
Content LanguagesEnglish
-
Upload UserAnonymous/Not logged-in
-
File Pages12 Page
-
File Size-